Evolution of analysis for nutrients in infant formula
Introduction
Breast milk is recognised as the best source of nutrition for infant growth and development, and is recommended by public health agencies.1 Under circumstances where breastfeeding may not be possible, unsuitable, or inadequate, infant formula is an effective substitute for infant feeding, as it is specially formulated to be a complete or partial substitute for human milk.2 For infants that are not breast-fed, infant formula is the sole source of nutrition in their first 6 months of life; as such it needs to meet all of their nutritional requirements. For this reason, infant formula is one of the most highly regulated food products in the world and is manufactured to rigorous specifications to maintain the highest product quality and safety. This paper briefly describes the development of infant formula products and the evolution of the analytical methods required to quantitate their nutrient content.
Development of infant formula
Archaeological evidence, based on the analysis of pottery residues, has dated the earliest consumption of ruminant milk by humans to around 7000 BCE. The history of alternatives to breastfeeding also goes back to ancient times, with evidence for the use of animal milk in human infant feeding around 2000 BCE. Before the invention of infant formula, infants could be fed by a wet-nurse (a woman who breastfeeds another’s child), or feeding milk from other mammals available: sheep, goats, camels, horses, or most commonly, cows.3 Providing optimal nutrition is critical during the first six months of infant life, and where breast milk was not accessible to the newborn, survival rates were extremely low.4
By the 19th century, developments in germ theory led to an emphasis placed on improvements in hygiene and in the quality of milk supplies. The invention of the rubber teat was a key breakthrough in feeding devices and significantly reduced health issues caused by microbial contamination of feeding bottles.4 This dramatic increase in survival rates led to an increase in demand and to the development of infant formulas as we recognise them today.
In the early- to mid-20th century, protein levels in cow milk-based infant formulas were systematically reduced and formulated to a whey:casein ratio comparable with that of human breast milk. The discovery of vitamins and their role in preventing diseases such as beriberi, scurvy, pellagra and rickets had a critical role in advancing the science of nutrition. As these key nutritional components became better understood, increasing focus was placed on developing formulas containing these constituents in appropriate ratios consistent with human milk.5 Towards the end of the 20th century, other non-vitamin micronutrients such as taurine, long-chain polyunsaturated fatty acids, and nucleotides became recognised as nutritionally important ingredients and are now routinely added.
Additionally, the diverse range of infant formula types on the market has expanded considerably. These different formulas have been designed to meet the differing needs of many infants and include those based on cows’ milk protein (suitable for the majority of infants), partially hydrolysed protein (marketed as being easier to digest, as they contain proteins that are partially hydrolysed), amino acids (offered to infants with cows’ milk protein allergy as they contain no intact protein), soy protein (suitable for infants where an alternative to cows’ milk formula is required) and low-lactose or lactose-free alternatives (intended for infants who are lactose intolerant).
New Zealand is a major exporter of dairy products, including infant formula. Analytical testing ensures that the products meet international standards for quality, safety and composition, which helps to protect the health of consumers and maintain the reputation of New Zealand’s dairy industry. Overall, dairy testing helps to ensure the competitiveness and sustainability of New Zealand’s dairy industry. The work undertaken daily at our nation’s manufacturing and contract laboratories is a vital, but often overlooked, component of food production in New Zealand.
Nutrients
A nutrient is considered an essential dietary component if it serves an indispensable physiologic function but cannot be synthesised endogenously at an adequate rate by healthy subjects.6 Vitamins play essential roles in human metabolism and are essential dietary components as they cannot be synthesised by the body, with the notable exception of vitamin D, making dietary sources critical. A range of other nutrients can usually be synthesised in adequate amounts endogenously; however, they may require exogenous supplementation under some circumstances. Vitamins and micronutrients play a fundamental role in an array of biochemical reactions for growth, development, health and reproduction with only trace amounts needed to maintain homoeostatic regulation of body function.
Fat-soluble vitamins (A, D, E, K) are stored in the body’s cells and are not readily excreted; excess fat-soluble vitamins are stored in the liver and adipose tissue and can accumulate to toxic levels if taken in excess. Water-soluble vitamins (B-group, C) are readily absorbed into tissues for immediate use and not stored in the body. They are quickly excreted in urine and will rarely accumulate to toxic levels; they therefore need to be regularly replenished from the diet.
Conditionally essential micronutrients are organic compounds that are ordinarily produced by the body in amounts sufficient to meet its physiological requirements; however, under stressful conditions their biosynthesis may be inadequate. Examples include choline, carnitine, inositol, nucleotides and taurine. The lack of overt symptoms of nutritional deficiency suggests that dietary sources provide adequate amounts of vitamins and minerals; however, there is concern that subclinical deficiencies may contribute to chronic diseases. These concerns emphasise the importance of nutrient-dense foods in the diet and recognition of the need for nutritional supplementation for individuals at risk for deficiency.7 Infant formula fortification with choline, carnitine, nucleotides and taurine to levels comparable with those in human milk is therefore advocated.
As well as formulating infant formula to mimic breast milk in content of macro and micronutrients, recent innovations and optimisation of infant formula composition have focussed on the addition of novel bioactive ingredients and their potential to provide additional health benefits for infants. Specifically, recent research has highlighted the importance of many other non-vitamin nutrients that may confer a range of health benefits and includes phospholipids, lactoferrin, oligosaccharides and stereospecific triglycerides.
Methods of analysis
A characteristic of micronutrients in infant formula is their relatively low concentration in a complex matrix of fat, protein and carbohydrates. Added analytical complexities arise due to the multitude of different forms, either exogenous or endogenous, in which a micronutrient may be found, and their relative instability to heat, light, oxidation, acids and alkali under analytical conditions. Analytical procedures that may be optimal for the removal of fat, protein and carbohydrates as well as other minor interfering components can be detrimental to the stability of the micronutrient of interest, making extraction and analysis all the more challenging.
Infant formulas are the most regulated food products and are rigorously tested for compliance with relevant national and international regulations. The nutrient composition of these products is designed to meet criteria for safety and suitability to minimise the risk of deficit or excess. This is critically important considering the vulnerable population these products serve. Testing these products is complicated due to their complex formulations and stability, so having standardised and validated methods is crucial to ensure testing is performed in a reproducible and reliable manner.
In developing the analytical methodology, consideration needs to be given to whether the goal is quantitation of the total vitamin including both endogenous and exogenous forms for nutritional purposes, or the selective quantitation of exogenous forms for labelling and compliance purposes (Table 1).8
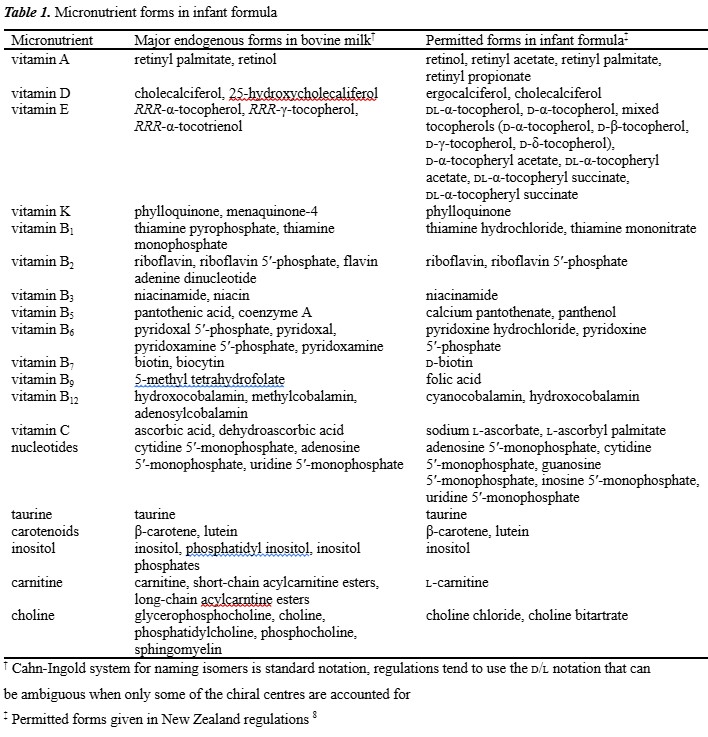
Fat soluble vitamins
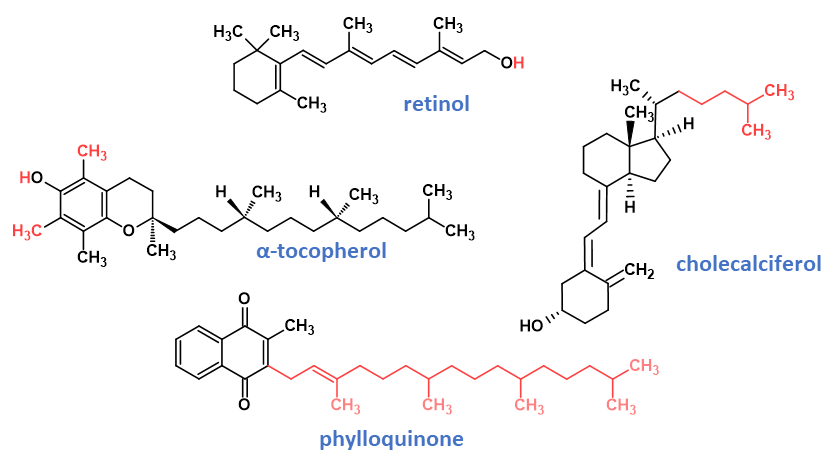
Vitamin A
Two groups of compounds possess vitamin A activity: retinol (Fig. 1) and its derivatives that are found naturally in animal products, and β-carotene and certain other carotenoids found in plants, which act as provitamin A sources. Vitamin A is typically fortified as retinyl esters, which are more stable than the parent retinol form. Provitamin A sources also contribute, although to a much lesser degree due to much lower retinol activity. Vitamin A supports cell growth, immune function, and foetal development. Perhaps one of the best-known functions of vitamin A is its role in vision and eye health in which 13-cis-retinal combines with the protein opsin to form rhodopsin, a molecule necessary for colour and low light vision.9
Methodologies for analysis of vitamin A and the carotenoids include colorimetry, spectrophotometry, fluorometry, paper, open-column and thin-layer chromatography, high performance liquid chromatography (HPLC) and capillary electrophoresis. Because of its significant molar absorptivity and fluorescence, vitamin A is a relatively straightforward vitamin for chemical analysis. An early method was the Carr-Price blue colour spectrophotometric method, introduced in 1926, but now superseded by modern strategies.10 In foods and infant formula, analysis of the vitamin is now typically achieved by saponification of ester forms to retinol, followed by extraction into organic solvent, with determination of vitamin content achieved by chromatographic separation and UV and/or fluorescence detection.9
Vitamin E
Vitamin E refers to a group of four naturally occurring tocopherols and four tocotrienols distinguished by the degree of methylation on the chromanol ring structure, and the degree of saturation of the isoprenoid sidechain. The important forms of vitamin E nutritionally are the naturally occurring RRR-α-tocopherol (Fig. 1), and the supplemented synthetic racemic all-rac-α-tocopherol, all-rac-α-tocopheryl acetate and all-rac-α-tocopheryl succinate. In many regulations, the contributions of β-, γ-, or δ-tocopherol and the tocotrienols are not relevant nutritionally but of importance only in terms of their contribution as antioxidants.
Vitamin E is readily oxidisable and the use of antioxidants throughout assays is common. In the harsh saponification environments used for high fat samples, antioxidants are mandated with pyrogallol, ascorbic acid, or butylated hydroxytoluene prevalent.9 A common early method first published in 1938 for vitamin E analysis known as the Emmerie-Engel method depended on tocopherol reduction of iron (III) to iron (II) and formation of a red complex with α,α-dipyridine. HPLC has long since replaced chemical methods for infant formula analysis, with sample preparation following a similar approach to that of vitamin A; that is, saponification of ester forms to α-tocopherol followed by extraction into organic solvent. Determination of vitamin content can then be achieved by chromatographic separation, most commonly reversed phase, with subsequent UV or fluorescence detection.9,10
Vitamin D
Vitamin D is a secosteroid in which a bond (C9–C10) in ring B of the steroid structure is broken. Nutritionally important forms of vitamin D are the vitamers ergocalciferol (vitamin D2) and cholecalciferol (vitamin D3; Fig. 1), and their respective 25-hydroxy metabolites. Ergocalciferol is synthesised by the photolysis of plant sterols, whereas cholecalciferol is synthesised in the epidermis of animals when exposed to UVB radiation from 7-dehydrocholesterol precursor that is converted to previtamin D3, which is spontaneously thermally isomerised to vitamin D3. Vitamin D is critical to promoting intestinal calcium absorption and maintaining adequate blood levels of calcium and phosphorus, both crucial for bone mineralisation. Ongoing research supports the importance of vitamin D in cancer, heart disease, type 2 diabetes, and autoimmune diseases. Alarmingly, the incidence of global vitamin D deficiency is reported to be increasing significantly since the 1980s, attributed to the increased use of sunscreens and decreased exposure to sunlight.
Vitamin D analysis is challenging for a number of reasons. As vitamin D exists in thermal equilibrium with the previtamin conformer, the simultaneous measurement of vitamin D and previtamin D is necessary to obtain an accurate and reliable estimate of its content in foods. Vitamin D is present in relatively low concentrations in complex food matrices, which can make analysis difficult due to the need for high sensitivity and selectivity to obtain sufficient signal and avoid interference from other components. Vitamin D can be sensitive to light, heat and oxygen and can degrade over time. This can make it challenging to ensure that the vitamin D content in food products is stable and that the analysis reflects the actual amount of vitamin D present in the product.
The determination of vitamin D in food matrices was traditionally accomplished using animal bioassay methods. However, these kinds of historical techniques are no longer in use. Since the 1980s, HPLC techniques have become the dominant approach to this analytical challenge since they facilitate the selective separation and analysis of each vitamin D form liberated during prior saponification and extraction. Indeed, for many years the official reference methods for determining vitamin D3 and vitamin D2 in foods have been based on normal-phase semi-preparative HPLC fractionation followed by reversed-phase LC-UV quantitation.11 More recently, given the attributes of MS detection, LC-MS methods have become increasingly prevalent, as is discussed later.
Vitamin K
The term vitamin K describes a group of naphthoquinones, some of which possess a terpenoid sidechain in the 3-position and exhibit antihaemorrhagic activity. The simplest form of vitamin K is menadione (vitamin K3) and is a synthetic form of the vitamin used in animal-feed supplements; however only phylloquinone (vitamin K1; Fig. 1) is used to supplement infant formula. Phylloquinone is produced by plants, while menaquinones (MKn, vitamin K2) containing unsaturated side-chains with varying chain lengths are, with the exception of MK4, products of bacterial synthesis, mainly by intestinal microflora.
In addition to its relatively low concentration, the analysis of vitamin K is made difficult due to its sensitivity to light and its susceptibility to degradation at high pH. Sample preparation steps for vitamin K analysis must therefore be undertaken in low-light conditions, and in the analysis of high fat foods such as dairy products, lipase digestion is commonly used as a benign alternative to alkaline saponification. Many early spectrophotometric chemistry methods were suitable only for very high-level vitamin K preparations, pure vitamin K preparations, concentrates and pharmaceuticals. The use of LC with UV, fluorescence (Fl) and electrochemical detection provided incremental benefits to vitamin K analysis in complex matrices. Vitamin K does not naturally fluoresce, although fluorescence can be induced if the quinones are reduced to the corresponding hydroquinone. HPLC methods were improved dramatically for routine deployment through the use of zinc post-column reduction, providing excellent recovery without the need for either wet chemical reduction or a second pumping system. Variations of this technique have become the standard for the analysis of vitamin K in infant formula and other dairy products.12
Water soluble vitamins
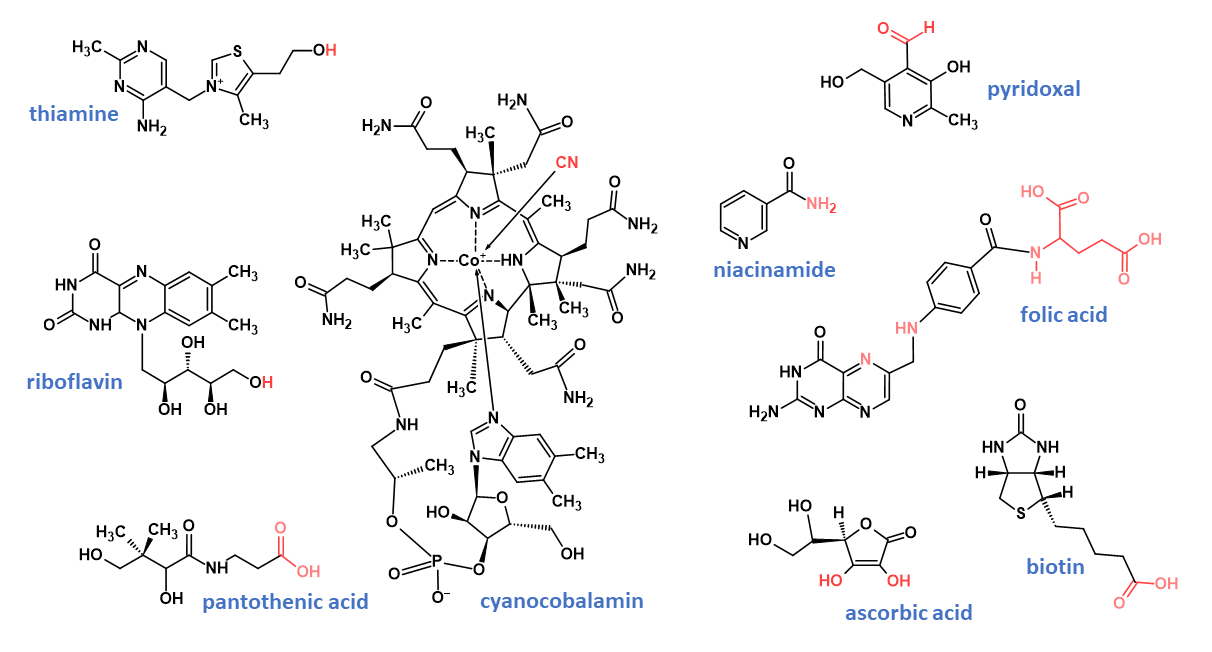
Vitamin B1
The structure of vitamin B1 consists of a thiazole ring joined by a methylene link to a pyrimidine ring; vitamers consist of thiamine (Fig. 2) and its phosphorylated derivatives. Vitamin B1 is an essential micronutrient that serves as a metabolic cofactor in several enzymatic reactions. Thiamine-dependent enzymes are important for the biosynthesis of neurotransmitters and reducing substances used in oxidant stress defences, as well as for the synthesis of pentoses used as nucleic acid precursors.13
The thiochrome reaction has been used since its development in 1935 for analysis of thiamine from biological matrices. The test procedure consists of sample extraction, enzymatic digestion, ion exchange clean-up, with an aliquot of the purified extract oxidised with 1% potassium ferricyanide in 15% sodium hydroxide. Thiochrome is then extracted with isobutanol and quantitated by fluorescence, with signal intensity proportional to the total thiamine in the sample, provided there are no other fluorescent compounds present.
Although this method is still used in some laboratories and remains an official AOAC method, modern alternative LC-Fl and LC-MS methods are now preferred and have largely replaced it.9,10
The reduction in analysis time with the advent of UHPLC coupled with specificity of tandem mass spectrometry (MS/MS) allows for routine analysis of multiple analytes simultaneously, often with enhanced method performance than earlier single analyte HPLC-UV methods. AOAC 2015.14, for example, specifies a method for the simultaneous quantitative determination of four water-soluble vitamins in infant formula and related nutritional products, including relevant forms of vitamins B1, B2, B3 and B6 by enzymatic digestion and UHPLC-MS/MS. However, LC-Fl is a less expensive technique and is still commonly used where vitamin B1 analysis only is required.14
Vitamin B2
Vitamin B2 belongs to the class of organic compounds known as flavins, with a structure characterised by an isoalloxazine tricyclic ring and containing a side chain attached to the N9 atom. Vitamin B2 as riboflavin (Fig. 2) is the key building block for its co-enzymatic forms flavin adenine dinucleotide (FAD) and flavin mononucleotide (FMN) that serve as electron carriers in various redox reactions involved in energy production and metabolic pathways, including carbohydrate, lipid and protein metabolism.9
Riboflavin is bright yellow and has a high degree of natural fluorescence when excited by UV light, a property that can be utilised conveniently in paper and TLC analyses.15 Rat and chick assays were developed for the analysis of riboflavin in the early 1930s, although these were mostly replaced in the next decade by microbiological analysis, followed by fluorometry in the 1970s. Routine LC-Fl assays were developed in the 1980s for the determination of free riboflavin as well total vitamin B2 including FAD and FMN, with fluorescence detection providing sensitivity and selectivity advantages compared with UV detection.9
While LC-MS methods are increasingly being used in infant formula analysis, LC-Fl is a less expensive technique and is still commonly used where vitamin B2 analysis only is needed.
Vitamin B3
Vitamin B3 includes the vitamers niacinamide (Fig. 2) and niacin. In the blood, brain, kidney and liver, vitamin B3 is converted to the coenzymes nicotinamide adenine dinucleotide and nicotinamide adenine dinucleotide phosphate, both of which are involved in the generation of energy in cells.
The classical König reaction was first applied to niacinamide in 1943, in which the oxidation of niacinamide by cyanogen bromide to the corresponding aldehyde, followed by coupling with a base like aniline, gives coloured products. A variety of methods based on microbiological assay by Lactobacillus plantarum has also been used.16 While these techniques are still occasionally used and are AOAC Official Methods, LC-UV methods are now prevalent due to their superior sensitivity and selectivity.9 Although multi-analyte LC-MS quantitation of vitamin B3 in conjunction with other B-vitamins is becoming prevalent, single-analyte testing of vitamin B3 is still commonly achieved by LC-UV.
Vitamin B5
Vitamin B5 vitamers include pantothenic acid (Fig. 2) and pantothenol that are key precursors for the biosynthesis of coenzyme A, a universal and essential cofactor involved in many metabolic reactions, including the synthesis of phospholipids, the synthesis and degradation of fatty acids, and the operation of the citric acid cycle.
Following the discovery of pantothenic acid as a vitamin in 1933, a number of microbiological methods (MBA) have been developed utilising many different microorganisms, with those based on L. plantarum most prevalent since it yields satisfactory recovery and superior precision to that obtained with most other microorganisms. This method uses a combination of enzymatic treatments to liberate pantothenic acid from its bound forms; however, its specificity is limited when dealing with complex food matrices. Alternative biochemical and physico-chemical methods have been developed to overcome limitations of MBA including spectrometry and fluorometry, radioimmunoassay and enzyme linked immunosorbent assay (ELISA), although reliable chromatographic methods offer advantages compared with these other methods in ease of use without the need for derivatisation, rapid analysis times and good precision.17 While LC-UV methods can be easier and less time-consuming, a major disadvantage is that detection of pantothenic acid requires UV wavelengths around 200 nm, a wavelength common to many other compounds present in samples. In addition, the absorptivity of pantothenic acid is low at this wavelength and as complete separation of pantothenic acid from other compounds is extremely difficult, interference free chromatography can be a challenge.18
An alternative biosensor immunoassay technique for the analysis of pantothenic acid, biotin, folic acid and vitamin B12 in dairy products is based upon surface plasmon resonance (SPR) detection coupled with vitamin-specific binding proteins.19 The specificity of the interaction minimises the need for lengthy and complex sample preparation steps common to microbiological assay and chromatographic methods. However, despite its many advantages, SPR-based immunoassays have largely been superseded in favour of LC-MS methods.
Vitamin B6
Vitamin B6 vitamers include pyridoxine (Fig. 2), pyridoxamine, pyridoxal, and their respective-5′-phosphorylated forms. Vitamin B6 has many functions in various systems of the body including nervous system, immune system, lipid metabolism, gluconeogenesis, erythrocyte function, hormone modulation and gene expression.
MBA methods have been used extensively for vitamin B6 analysis, although this is challenging as the ideal organism would show equal growth response for all the different forms of vitamin B6. This is not always the case, although Saccharomyces carlsbergensis is widely used as it responds well to all three free bases. Similarly, an ideal ELISA method broadly specific to all forms and with application to the measurement of total B6 would be desired; however, no such method exists.20
Routine chromatographic analysis of total vitamin B6 typically involves hydrolysis of phosphorylated forms followed by reversed-phase chromatography with fluorescence detection. In general, LC-UV methods either quantify the three forms separately, or chemically transform to a single vitamer form to determine an aggregate total vitamin B6. In fortified products where the fortified free form dominates, this analysis is straightforward, and the hydrolysis step is omitted.
As with other B-vitamins, the LC-MS platform is increasingly used for multi-analyte testing, while vitamin B6 alone is still commonly tested by the simpler LC-UV technique.
Vitamin B7
Biotin (Fig. 2) and biocytin are the main vitamers of vitamin B7 and are necessary components for the function of several carboxylase enzymes that are part of important metabolic processes including the production of glucose and fatty acids. There are many challenges when analysing biotin in complex matrices such as infant formula. Biotin is found in very low concentrations, making it difficult to detect and measure accurately. Biotin is often bound to proteins and other biomolecules, making it difficult to separate from other components in a sample. As it is a water-soluble vitamin, organic solvent for the extraction cannot be used to simplify extraction.
Numerous methodologies in the analysis of biotin take advantage of extremely tight binding between biotin and avidin. Strategies based on this binding affinity include fluorescence, chemiluminescence, bioluminescence, radiometric assays, ELISA and electrochemical assays.21
Biotin has no significant chromophore and does not fluoresce, making detection difficult and requiring extensive sample clean-up prior to instrumental analysis. Both pre-column and post-column derivatisation strategies have been used to overcome this limitation. LC-MS analysis now offers the ease of chromatographic separation of biotin without the limitations of UV detection.
Vitamin B9
Folic acid (Fig. 2), the most stable, fully oxidised vitamin B9 (folate) vitamer, is used for fortification of foods. Other naturally occurring vitamers include numerous reduced forms such as 5-formyltetrahydrofolate, and 5-methyltetrahydrofolate. Folate plays a key role in red blood cell formation and is critical to healthy cell growth and function. During early pregnancy, folate is crucial to reduce the risk of birth defects of the brain and spine.
Naturally occurring reduced folates are labile under a variety of conditions including heat, light, acids and oxidation and are present at relatively low concentration in a range of reduced states and polyglutamate forms. Turbidimetric MBA methods based on Lactobacillus casei were commonly used to determined folate. For biological and chromatographic methods, it is common to convert polyglutamate forms to the mono- or di-glutamate forms with a deconjugase enzyme prior to further analysis. A common sample preparation approach, taken for many foods, is to use an initial tri-enzyme digestion strategy in which an amylase and a protease are used to release bound folates.22
The selective determination of folic acid in fortified food products, where folic acid is the dominant form, is more facile, facilitating the routine use of LC-UV and LC-MS techniques available for rapid reporting for compliance testing, without the need for multi-enzyme digestions.
Vitamin B12
Vitamin B12 vitamers include methylcobalamin, adenosylcobalamin, hydroxocobalamin, and cyanocobalamin (Fig. 2). As the most stable form of vitamin B12, synthetic cyanocobalamin is the form typically used as a food supplement and is converted into physiologically active adenosylcobalamin and methylcobalamin forms in the body.
Historically, determination of cyanocobalamin in foods was performed using an MBA with Lactobacillus leichmannii. Although MBA are highly sensitive for vitamin B12, the microorganism may metabolise non-cobalamin corrinoids which have no vitamin activity and hence potentially overestimate vitamin B12 content. The MBA method is time consuming and less precise as compared with chromatographic methods.23
Vitamin B12 analysis in foods is typically performed after the release of protein-bound forms, followed by heat treatment and conversion to the cyano-form in the presence of cyanide ions. Handling of cyanide reagent carries risk due to its acute toxicity; however, these risks can be mitigated to a large extent with a robust health and safety programme. Due to low UV absorptivity and low abundance, LC-UV analysis of vitamin B12 is challenging, therefore pre-concentration of cyanocobalamin in the sample extract using either reversed-phase or immunoaffinity cartridges is common. Detection at absorption maxima at 361 and 550 nm eliminates UV contribution from many potential interferences in a food sample.
The use of inductively coupled plasma-MS coupled to capillary electrophoresis or LC for the indirect determination of cyanocobalamin by quantifying cobalt in the cobalamin molecule has been reported, providing a facile approach for the determination of vitamin B12.
Vitamin C
Vitamin C exists in two redox states, l-ascorbic acid (Fig. 2), and its oxidised form, l-dehydroascorbic acid. Vitamin C is an essential factor for the growth, development and repair of all body tissues and is involved in many body functions, including formation of collagen, absorption of iron, the proper functioning of the immune system, wound healing, and the maintenance of cartilage, bones and teeth. Unlike most vertebrates, primates including humans, lack the ability to synthesise vitamin C from glucose. Hence, exogenous vitamin C is required for the prevention and treatment of vitamin C related deficiency.
In general, the analysis of ascorbic acid in food is challenging, primarily due to both its low oxidative stability with facile conversion to dehydroascorbic acid and potential matrix interferences. Throughout the analysis, preventive steps are therefore mandatory to minimise analyte degradation. Stabilisers and/or chelators (meta-phosphoric acid, trichloroacetic acid, homocysteine, trifluoroacetic acid, oxalic acid and EDTA) are therefore often added prior to sample storage or preparation. Many analytical methods have been reported to measure vitamin C content in foods. Despite its limitations, classical titrimetric methods are still common in milk and infant formula analysis due to simplicity and low cost.
Because of the polar nature of vitamin C, hydrophilic interaction liquid chromatography (HILIC) is increasingly becoming the preferred chromatographic technique, although ion exchange, ion-pair, ion-exclusion and reversed-phase liquid chromatography (RPLC) have also been reported. Ascorbic acid and dehydroascorbic acid are poorly retained on RPLC columns, requiring a low pH to both avoid degradation and to facilitate retention. The absorbance maximum of ascorbic acid is in the range of 244 to 265 nm as a function of pH, whereas the detection of dehydroascorbic acid requires prior derivatisation due to its poor UV absorption. LC-MS has been rarely used, due to matrix effect-induced signal suppression necessitating the inclusion of stable isotope-labelled internal standards for reliable quantitation, and the incompatibility of MS with the commonly utilised eluent additives.
Conditionally essential nutrients
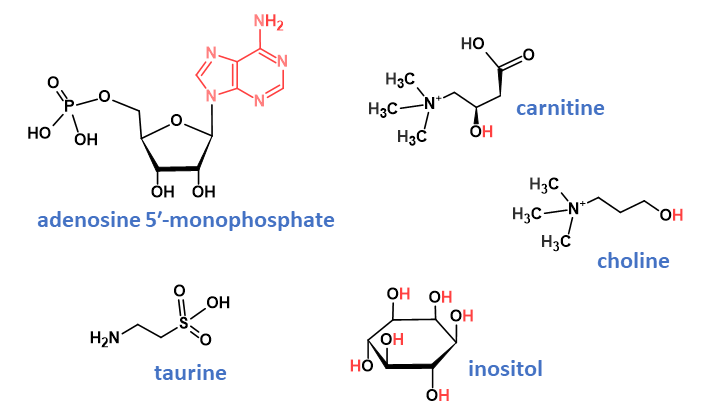
Nucleotides
Nucleotides are building blocks of nucleic acids, the carriers of genetic information. Fortification of foods is implemented with adenosine 5′-monophosphate (Fig. 3), guanosine 5′-monophosphate, inosine 5′-monophosphate, cytidine 5′-monophosphate, and uridine 5′-monophosphate. Nucleotides have many important physiological and biochemical functions and have been considered as non-essential as they can be synthesised by the body without the need for supplementation. However, exogenous nucleotides can be readily absorbed and utilised, reducing the body’s need for de novo synthesis or salvage. Recent studies have found that exogenous nucleotides are indispensable nutrients for specific physiological conditions, including when immune-compromised, if malnutrition exists or during periods of rapid growth or aging.24
Sample preparation of infant formula is generally achieved through removal of proteins by either acidic precipitation or centrifugal ultrafiltration and further clean-up with either ion-exchange solid phase extraction or phenylboronate affinity gel. RPLC with and without the addition of ion-pair reagents, ion-exchange LC and hydrophilic interaction liquid chromatography (HILIC) with UV detection are commonly used for the quantitation of nucleotides in milk products. The use of MS offers potential advantages by incorporating stable isotope labelled internal standards, while the selectivity of tandem mass spectrometry (MS/MS) reduces the need to remove other components that often compromise UV analyses.25
Carnitine
l-Carnitine (Fig. 3) is synthesised from the amino acids lysine and methionine and is required for mitochondrial β-oxidation of long-chain fatty acids for energy production. Long-chain fatty acids must be esterified with carnitine, yielding acylcarnitines, to enter the mitochondrial matrix where β-oxidation occurs.26
Many analytical methods have been reported for the separate determination of either free or total carnitine in milk, infant formula, clinical tissues and foods, predominantly by enzymatic, electrophoretic or chromatographic techniques, although biosensor techniques have also been described. Conventional LC is limited, and prior analyte derivatisation and/or ion pair reagents are used to enhance retention and detection response under reversed-phase conditions. Until recently, enzymatic methods have most commonly been routinely applied for infant formula compliance purposes and although successful, are manipulative, time consuming and require separate determinations.26
Choline
Choline (Fig. 3) is a quaternary amine containing two carbons and a hydroxyl group and is a critical precursor in the maintenance of the phospholipid structure of cell membranes. Choline is also a component of lipoprotein phospholipids, in phospholipids for cell signalling molecules such as platelet activating factor and is a precursor of the neurotransmitter acetylcholine.26
Due to the similar structural and chemical properties of carnitine and choline, similar analytical approaches are commonly implemented to measure free or total choline in infant formula, with enzymatic, electrophoretic or chromatographic techniques all used. With the proliferation of LC-MS/MS platforms, and due to their similar molecular structures and physicochemical properties, this technique has increasingly been exploited for the simultaneous determination of both free and total choline and carnitine contents in foods, utilising reversed-phase, HILIC or strong cation-exchange column separation modes. For regulatory compliance of paediatric formula foods, sample treatments utilised in these methods are generally optimised for the quantitation of total carnitine and total choline, since analyte speciation is not required. A direct comparison of individual enzymatic and simultaneous LC-MS/MS methods for the determination of total carnitine and choline in milk and infant formula products have been reported.27
Inositol
myo-inositol (Fig. 3) is a six-carbon polyol where each carbon is hydroxylated. It plays an important role in various cellular processes as the structural basis for secondary messengers in cells such as inositol triphosphates and phosphatidylinositol lipids. Inositol is important or even essential in a wide range of cell functions, including cell growth, development and functioning of peripheral nerves, osteogenesis and reproduction. Milk is a significant source of myo-inositol, both free and bound, and since human milk contains higher levels of myo-inositol than bovine milk, it is commonly added to infant formulae to ensure against potential early neonatal deficiency.26
The analysis of free myo-inositol in foods, including dairy products, has generally been facilitated following protein precipitation with dilute acid, whereas methods targeting the sum of free and bound forms have followed an exhaustive, highly concentrated acidic or alkaline hydrolysis at high temperature. Analytical detection and quantitative techniques have included microbiological assay, enzymatic assay, gas-liquid chromatography with prior derivatisation and HPLC coupled to either UV, MS, or evaporative light scattering detection (ELSD). In view of the electrochemical properties of myo-inositol, high performance anion-exchange chromatography (HPAEC) coupled to pulsed amperometric detection (PAD) has recently become increasingly recognised as a highly sensitive and specific platform for its determination in foods, infant formula and species milk in both free and/or bound forms.28
Taurine
Taurine (Fig. 3), 2-aminoethanesulfonic acid, is a β-amino acid; unlike other amino acids, however, it is not found within protein structures. Although taurine is an essential nutrient for infants, adults are able to synthesise it and in general, obtain sufficient taurine from the diet. Taurine supports digestion through the absorption of fat from the gastrointestinal tract, maintains liver function and functions within the nervous system where it regulates calcium and controls inflammation.
Taurine is easily extracted from milk, milk powders and infant formulations. As it is a small molecule without a chromophore, its detection requires comparable derivatisation or electrochemistry techniques as for other amino acids. Taurine is readily analysed in the same manner as traditional amino acid profiles using ion-exchange columns to separate underivatised amino acids, followed by post-column ninhydrin reaction in discrete amino acid analysers. Such devices are still useful but they have largely been superseded by HPLC instrumentation techniques that are more versatile. Alternatively, the analysis of taurine can be accomplished using a pre-column dansyl chloride derivatisation reaction and reversed-phase LC-UV or LC-Fl. Despite the selectivity advantages of LC-MS, quality assessments of taurine in most infant formulas are probably adequately provided by existing reversed-phase HPLC techniques, although LC-MS is advantageous when analysing matrices in which taurine is not easily discriminated, such as protein hydrolysates.26
Additional nutrients
New formulas are created to satisfy the nutritional requirements of infants who have specific nutritional requirements. Advances continue to be made, resulting in infant formula products with compositions and functions closer to those of human milk (Table 2).
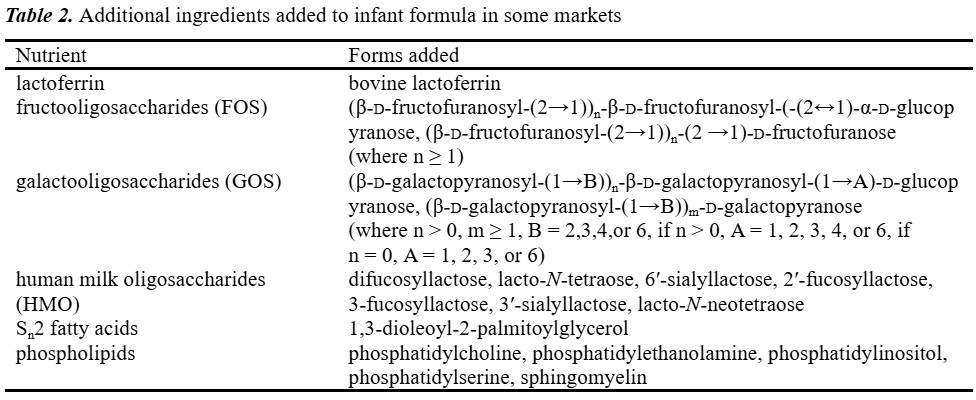
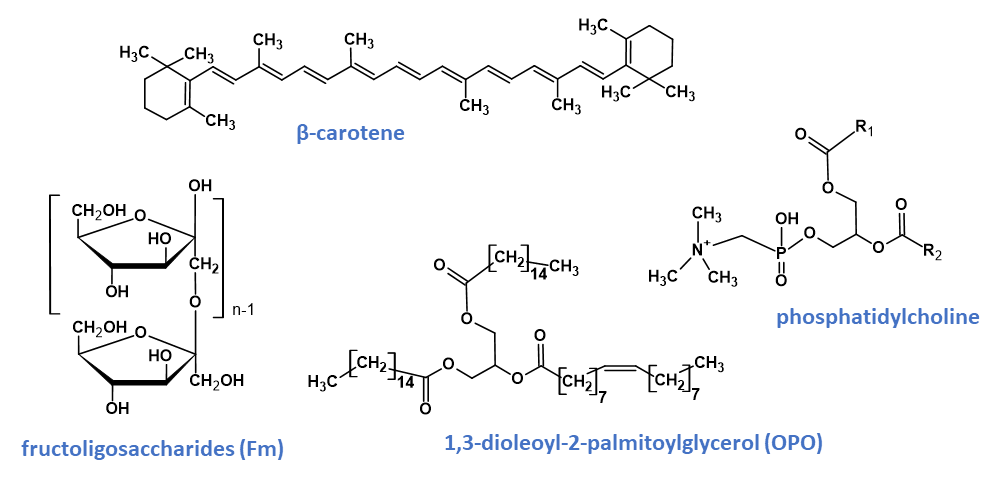
Carotenoids
Carotenoids typically have a 40-carbon chain backbone composed of eight isoprene moieties. Carotenoids are efficient scavengers of reactive oxygen species, especially singlet molecular oxygen and peroxyl radicals, helping in disease protection and enhancing the immune system. Provitamin A carotenoids can be converted in vivo into vitamin A, which is essential for growth, immune system function and eye health. Carotenoids are composed of two or more units of hydrocarbons and are categorised into two sub-groups known as carotenes and xanthophylls. Carotenes [β-carotene (Fig. 4), α-carotene, and lycopene] are made up entirely of carbon and hydrogen, whereas xanthophylls (β-cryptoxanthin, lutein, astaxanthin and zeaxanthin) also contain oxygen. The carotenoids are highly unstable and degrade rapidly under several conditions such as heat, oxygen, light, moisture and certain pH.
Carotenoid analysis generally encounters many difficulties due to chemical instability, intra- and inter-group chemical heterogeneity, low concentration and matrix effects as well as interaction with other food components. Most commonly, carotenoids are extracted via alkaline saponification followed by liquid-liquid extraction using organic solvents. Since this approach may induce degradation, alternative strategies such as enzymatic lipolysis and compressed fluid, supercritical fluid and miniaturised extraction techniques have also been reported.
Although reversed-phase LC platforms utilising either C18 or C30 column chemistries are typically used in carotenoids analysis, normal-phase separations have also been reported because of superior retention of polar xanthophylls and enhanced resolution of carotenoid isomers. LC platforms are commonly equipped with photodiode array detectors, as multi-channel UV is considered essential to distinguish between the individual carotenoids, although mass spectrometric detection is increasingly used for confirmation of identity.26
Oligosaccharides
Prebiotic oligosaccharides are the third most prevalent component in mothers’ milk. The addition of prebiotics in infant formula alters the gastrointestinal microbiota, resembling that of breastfed infants, with a lower stool pH, a better stool consistency and frequency and a higher concentration of intestinal bifidobacteria compared with infants on a non-supplemented standard formula. Galactooligosaccharides (GOS) and fructooligosaccharides (FOS) are polymers of galactose and fructose (Fig. 4), respectively, and although incorporated in infant formula, differ structurally from oligosaccharides naturally occurring in human milk.
Human milk oligosaccharides (HMO) contain lactose at their reducing end. N-acetylneuraminic acid and fucose, which are abundant constituents of HMO and may be important for some HMO functions, are not part of GOS or FOS. In contrast to cows’ milk, human milk contains non-lactose oligosaccharides in very high quantities. The complexity of HMO is also much greater than that observed for the oligosaccharides in the milk of most other mammals.
Following prior strategies to remove major milk components such as lipids, proteins and lactose, analysis of milk oligosaccharides commonly makes use of a separation technique coupled to a mode of detection. Identifying and quantifying the many closely related oligosaccharides in milk represent a major challenge due to the complexity of their structures. Separation is generally achieved by either reversed-phase, HILIC or anion-exchange chromatography (AEC) columns, or by capillary electrophoresis. To detect the oligosaccharides after separation, there is a choice between labelled (fluorescent or UV active labels covalently coupled to the oligosaccharide) and label-free detection (refractive index, ELSD, PAD and MS). It should be noted that without derivatisation, oligosaccharides cannot be detected with the most widely used optical detection methods due to their poor UV absorption and lack of fluorescent characteristics. HPAEC-PAD is a well-established technique for the analysis of oligosaccharides that does not require derivatisation, although it is important to note that the detection sensitivity of this approach is orders of magnitude less than that of fluorescence or MS detection. HILIC with fluorescence detection of oligosaccharides derivatives is another frequently used analytical tool.29,30
The major challenges in undertaking oligosaccharide analyses in infant formula is that many of the oligosaccharide standards are not available commercially. In the absence of suitable standards, response factors need to be determined by interpolation, or by evaluating oligomer profiles of the relevant GOS or FOS raw material.26 Furthermore, the long chromatographic run times of typically 30–60 min is a limitation to high throughput, routine analyses.
Phospholipids
A phospholipid contains two hydrophobic tails of fatty acids and one hydrophilic head of a phosphate moiety joined together by an alcohol or glycerol molecule. Phospholipids can be categorised as glycerophospholipids and sphingomyelins. Glycerophospholipids contain a glycerol backbone and are the main type of phospholipids in eukaryotic cells. Based on the variation in the type of hydrophilic head group, glycerophospholipids can be further subclassified into phosphatidylcholine (Fig. 4), phosphatidylethanolamine, phosphatidic acid, phosphatidylserine, phosphatidylinositol and phosphatidylglycerol. Sphingomyelins contain a sphingosine backbone and are an integral part of the lipid bilayer of animal cell membranes. Phospholipids have been used widely in the food industry as emulsifiers but it is their nutritional function in enhancing cognitive development and immune support that has been the focus of nutrition research over recent years.
Milk polar lipids are difficult to comprehensively analyse, which limits the investigation of their functions. Commonly reported methods for phospholipids analysis are 31P NMR and LC-ELSD. However, these methods can only distinguish individual subclasses, but cannot speciate the composition of phospholipids within a subclass. 31P NMR spectroscopy can facilitate simultaneous qualitative and quantitative analyses of all phospholipids with only one internal standard. However, despite its many advantages, high-field NMR spectroscopy is an expensive technique, thus LC techniques are widely used as a routine method for quantifying phospholipids in foods.31
HILIC-MS is applicable for both qualitative and quantitative analysis of each phospholipid subclass. HILIC-MS shows good performance, with elution behaviour depending predominantly on polar head group hydrophilicity. Phospholipids species can be divided into their classes, which prevents the co-elution of phospholipids with similar m/z values but different head groups, thus reducing misidentification.
SN2 fatty acids
Structured triglycerides, in which palmitic acid has been predominantly esterified to the SN2 position and developed to mimic human milk fat, have been demonstrated to benefit fat and calcium absorption, bone mineralisation and strength, intestinal flora and infant distress in healthy infants.
The esters formed between glycerol and fatty acids produce a vast array of potential mono-, di- and triacylglycerides. Triacylglycerides, the predominant neutral lipid compounds, have three regiospecific positions for esterification (SN1, SN2 and SN3). Newer infant formulations are modified to include certain stereospecific triacylglycerides, in particular 1,3-dioleoyl-2-palmitoylglycerol (OPO; Fig. 4) such that the fatty acid profiles and the SN2 compositions become more closely aligned with those in human milk. The positions of the fatty acids have assumed greater importance in infant nutrition as triglycerides in which palmitic acid has been predominantly esterified to the SN2 position.
As an ingredient of infant formula, a method of differentiating OPO from the endogenous milk-fat triacylglycerides is required. A simplified approach to OPO analysis uses gas chromatography with flame-ionisation detection (GC-FID) to separate intact triacylglycerides based on carbon number (C52), but it is generally insufficient to discriminate OPO from other C52 triacylglycerides for quality-control purposes. A more targeted approach is to initially separate triacylglycerols chromatographically and then selectively trans-esterify the SN1/SN3 positions of the triacylglycerols using specific enzymes to yield 2-monoacylglycerols, which are subsequently separated chromatographically, and their fatty acid composition determined by GC-FID or LC-MS.32
Lactoferrin
Lactoferrin is a secretory glycoprotein characterised by carbonate-anion-dependent, high-affinity reversible binding of two Fe3+ ions per molecule. As a minor milk protein, lactoferrin exhibits a range of physiological properties that provide growth, immunoprotective and antimicrobial factors to the neonate and may function in the regulation of intestinal iron uptake, immune response, growth factor activity, bone growth and antioxidant activity. Lactoferrin is present in human milk at significantly higher levels than in bovine milk and isolated bovine lactoferrin is increasingly used as an ingredient in infant formula manufacture to enhance the nutritional efficacy of this food.
A variety of analytical techniques have been used in the analysis of lactoferrin in dairy products, including capillary electrophoresis, reversed-phase LC-UV, LC-MS and ELISA. An automated biosensor SPR-based immunoassay has been reported for the quantitation of lactoferrin in bovine milk and infant formula milk. This technique exploits the specific and reversible interaction between antibody and protein antigen providing a rapid and robust method for the quantitative analysis of lactoferrin in the presence of many other milk proteins.14, 26
Standards setting
AOAC International
AOAC International (est. 1884) is a US-based standards developing organisation that advances food safety, food integrity and public health, by bringing together members, organisations and experts dedicated to developing and validating reference methods; a key element to the objective of establishing “trueness” in analytical science. Through its Official Methods Program, fully validated and peer-reviewed methods are used by industry, regulatory agencies, research organisations, testing laboratories and academic institutions. The US Code of Federal Regulations recognises Official Methods of Analysis (OMA), and they are legally defensible in US courts as well as many other jurisdictions worldwide.
SPIFAN, the Stakeholder Program on Infant Formula and Adult Nutrition, is an integrated programme within AOAC International that evaluates, validates and approves test methods for infant formula and adult nutritional products. SPIFAN consists of working groups and expert review panels that meet regularly to discuss the changing needs of industry and regulators and facilitates the creation of new methods to test specific nutrients. A working group is tasked with the development of standard method performance requirements (SMPR), which is a set of guidelines defining the analyte scope, what forms are to be included or excluded, as well as the validation performance parameters that a method is required to meet to be considered as a possible standard reference method. Candidate methods are thoroughly validated and evaluated by peer-review to ensure their accuracy, precision and robustness, making them a reliable and accepted method of analysis for infant formula. During this phase of standard development, debate is robust and transparent, even occasionally blunt, but the process ensures only demonstrably fit-for-purpose methods are adopted.
As new technologies and analytical techniques are developed, AOAC continues to update OMA and publish new analytical methods to ensure that infant formula products continue to be reliable, safe and comply with quality control and regulatory requirements. Since its creation in 2010, SPIFAN has developed nearly 30 Final Action Official Methods intended for use internationally as dispute resolution reference methods, with equally as many selected as First Action methods from a vast pool of candidate methods (Table 3).

International Organisation for Standardisation
The International Organisation for Standardisation (ISO, est. 1947) is an international standard development organisation composed of representatives from the national standards organisations of member countries. While ISO establishes international standards, not all countries use the same standards for every aspect of their operations. Adoption of ISO standards can vary, depending on factors such as the specific industry, national regulations and local practices. Countries are not obligated to adopt ISO standards but many choose to do so because of the benefits they offer, including improved efficiency, enhanced safety and increased credibility in the global marketplace.
ISO and the International Dairy Federation (IDF) collaborate closely on all matters of standardisation relating to methods of analysis and sampling for milk and milk products, and since 2001 they have published joint standards using the logos and reference numbers of both organisations.
An agreement exists between AOAC and ISO to allow for joint development and approval of standards, which involves establishing working groups comprising AOAC and ISO experts to develop draft common standards. The standards are then subject to parallel approval processes, with completed standards published by both organisations. Since ISO membership consists of 167 countries, a key benefit of coordination between AOAC and ISO is that SPIFAN derived methods obtain the widest possible adoption and recognition by governmental regulators.
Codex Alimentarius Commission
The Codex Alimentarius Standard for Infant Formula and Formulas for Special Medical Purposes intended for infants (CXS 72-1981) together with the Codex Standard for Follow-Up Formula (CXS 156-1987) contain provisions for essential composition, quality and safety factors for those products and constitute the international reference, which is the basis for many countries’ national or regional regulations.
One of the key goals of the SPIFAN programme is to gain global acceptance of its methods. The SPIFAN methods have been reviewed for acceptance by the AOAC, ISO, and finally Codex with the intent to have them adopted as ‘Type II’ dispute resolution methods. With this designation, these methods will be the benchmark upon which other methods will be compared.
New Zealand’s contribution
Methods developed
Over the last quarter of a century, New Zealand scientists have been active in developing and validating international reference methods for nutrients analysis in infant formula and other dairy products. Although the New Zealand contingent to SPIFAN consists of only a handful of chemists, the number of methods developed in New Zealand accounts for 20% (6/30) of the output of international reference methods developed as part of the SPIFAN programme.
AOAC 2011.20 and ISO 20638 are a method for the determination of nucleotides (AMP, CMP, GMP, IMP and UMP) in infant formula developed at the Fonterra Nutritionals Laboratory.14 Following sample dissolution, potential interferences were removed by anion-exchange SPE, with the extract analysed by HPLC-UV. This is the first AOAC Official Method for these analytes and its ease of use has led to an expansion in the scope of matrices and its further adoption for other foods and animal feeds.
AOAC 2016.02 for biotin in infant formula was developed by AsureQuality, Auckland.14 The sample is dispersed in phosphate-buffered saline and then autoclaved. A filtered extract is cleaned up on an immunoaffinity column mounted to an SPE manifold. The biotin extracted is then quantified by HPLC-UV at 200 nm. The immunoaffinity chromatographic extraction is the key step to successful analysis, as it is specific for retention of biotin, allowing most other interfering components in the sample to be removed from the sample extract.
The latest official method for vitamin D, AOAC 2016.05 and ISO 20636, was first published in 2015 and was developed at the Fonterra Nutritionals Laboratory for the quantitative determination of vitamin D2 and vitamin D3 in infant formula and adult nutritionals.14 This LC-MS/MS method is a significant improvement over earlier LC-UV methods with improved sensitivity and specificity, which allows for more accurate, precise and reliable measurement of vitamin D levels. The method is also more efficient with a dramatic improvement in sample throughput over earlier official methods. The impact of this method has been significant, as it has helped to ensure the accuracy and reliability of vitamin D analysis in food products, particularly in infant formula. This is particularly important, as vitamin D is an essential nutrient for infants and young children; ensuring that infant formula provides the appropriate levels of this nutrient compliant with an extremely narrow regulatory specification range is a critical food safety test.
A real-time, label-free optical biosensor surface-plasmon resonance method for the quantitation of lactoferrin in bovine milk and infant formula milk was adopted as AOAC 2021.07.14 This method was developed in the Fonterra Nutritionals Laboratory in 2005 and has been used successfully for many years. Automated biosensor immunoaffinity technologies exploit the specific and reversible interaction between antibody and protein antigen and they are versatile, robust and capable of producing rapid and reliable data for the quantitative analysis of lactoferrin in complex food matrices with minimal sample preparation. This technique also offers the advantage of specifically detecting intact physiologically functional protein since denatured, inactive protein does not interact and is therefore excluded from lactoferrin measurement.
Most recently, a HILIC-MS/MS method for taurine in infant formulas developed at Fonterra was adopted as an AOAC 2022.03.14 HILIC is particularly advantageous when coupled to MS detectors, in that the high organic content of the mobile phase enhances spraying and desolvation, thereby increasing signal intensity; also, there is less need for gradient elution, thereby reducing the impact of variances in the composition of the mobile phase on ion suppression. This method completed the SPIFAN amino acid programme wherein all other amino acids had been successfully incorporated into official methods several years earlier.
Additionally, New Zealand scientists have been instrumental in the development of other reference methods for infant formula and other dairy products outside the scope of the SPIFAN programme or prior to its inception. These include AOAC 997.05 (an HPLC-UV method for the determination of taurine), AOAC 997.14 (an enzymatic method for determination of choline), AOAC 999.15 (an HPLC-Fl method for vitamin K), AOAC 2010.01 (the analysis of bovine immunoglobulin G by HPLC-UV), AOAC 2011.01 (a kit-based SPR-based automated immunoassay method for vitamin B12) and AOAC 2012.26 (an automated immunoassay method for determination of IgG in dairy powders).14
Summary
The efforts of New Zealand scientists have been critical to the success of the SPIFAN project in its quest to standardise methods of analysis for infant formula or other dairy products and gain international adoption by AOAC, ISO and Codex. In addition to the authors, several other scientists have made significant contributions in an advisory/managerial role, as method developers or as technical experts; these include Grant Abernethy, David Woollard, George Joseph and Steve Holroyd.
Further developments in the science of infant nutrition, the attendant improvements in product formulations and updates in regulations suggest that the SPIFAN project will continue into its second decade at the same rapid pace as it completed its first.
References
- Leung, A.K.; Sauve, R.S. J Natl Med Assoc 2005, 97, 1010–1019.
- Green Corkins, K.; Shurley, T. Nutr Clin Pract 2016, 31, 723–729.
- Stevens, E.E.; Patrick, T.E.; Pickler, R. J Perinat Edu 2009, 18, 32–39.
- Happe, R. P.; Gambelli, L. In Specialty Oils and Fats in Food and Nutrition (Ed.: Talbot, G.), Woodhead Publishing, Cambridge, 2015, 285–315.
- Wargo, W.F. J AOAC Int 2016, 99, 7–11.
- Chipponi, J.X.; Bleier, J.C.; Santi, M.T.; Rudman, D. Am J Clin Nutr 1982, 35, 1112–1116.
- Bridgeman, M.M.; Rollins, C.J. In Handbook of Non-prescription Drugs: An Interactive Approach to Self-Care (Eds.: Krinsky, D.L., Ferreri, S.P., Hemstreet, B.A., Hume, A.L.), APhA, Washington, DC, 20th ed. 2020, 285–315.
- Food Standards Australia New Zealand, Australia New Zealand Food Standards Code (Schedule 29—Special Purpose Foods), 2015.
- Eitenmiller, R.R.; Landen Jr., W.O.; Ye, L. Vitamin Analysis for the Health and Food Sciences, CRC Press: Boca Raton, FL, 2nd ed. 2007.
- Ellefson, W.C. J AOAC Int 2016, 99, 12–14.
- Staffas, A.; Nyman, A. J AOAC Int 2003, 86, 400–406.
- Fauler, G.; Muntean, W.; Keis, H.J. In Modern Chromatographic Analysis of Vitamins. (Eds.: De Leenheer, A.P.; Lambert, W.E.; Van Bocxlaer, J.F.), Marcel Dekker, New York, 3rd ed, 2000, 229–270.
- Tanphaichitr, V. In Handbook of Vitamins. (Eds.: Rucker, R.B; Suttie, J.W.; McCormack, D.B.; Machlin, L.J.), Marcel Dekker, New York, 3rd ed, 2001, 275–316.
- AOAC International, Official Methods of Analysis (Ed.: Latimer Jr., G.W.), Oxford Academic, New York, 22nd ed. 2023.
- Neilsen, P. In Modern Chromatographic Analysis of Vitamins. (Eds.: De Leenheer, A.P.; Lambert, W.E.; Van Bocxlaer, J.F.), Marcel Dekker, New York, 3rd ed, 2000, 391–433.
- Eitenmiller, R.R.; De Souza, S. In Methods of Vitamin Assay. (Eds.: Augustin, J.; Klien, B.P.; Becker, D.; Venugopal, P.B.), John Wiley & Sons, New York, 4th ed, 1985, 385–398.
- Wyse, B.W.; Song, W.O.; Walsh, J.H.; Hansen, R.G. In Methods of Vitamin Assay. (Eds.: Augustin, J.; Klien, B.P.; Becker, D.; Venugopal, P.B.), John Wiley & Sons, New York, 4th ed, 1985, 399–416.
- Velíšek, J.; Davídek, J. In Modern Chromatographic Analysis of Vitamins. (Eds.: De Leenheer, A.P.; Lambert, W.E.; Van Bocxlaer, J.F.), Marcel Dekker, New York, 3rd ed, 2000, 555–600.
- Gao, Y.; Guo, F.; Gokavi, S.; Chow, A.; Sheng, Q.; Guo, M. Food Chem 2008, 110, 769–776.
- Ball, G.F.M. Water-Soluble Vitamin Assay in Human Nutrition, Chapman & Hall, London, 1994.
- Ploux, O. In Modern Chromatographic Analysis of Vitamins. (Eds.: De Leenheer, A.P.; Lambert, W.E.; Van Bocxlaer, J.F.), Marcel Dekker, New York, 3rd ed, 2000, 479–510.
- Arcot, J.; Shrestha, A. Trends Food Sci Technol 2005, 16, 253–266.
- Martin, F.; Campos-Giménez, E.; Konings, E. J AOAC Int 2016, 99, 19–25.
- Gill, B.D.; Indyk, H E. J AOAC Int 2007, 90, 1354–1364.
- Gill, B.D.; Indyk, H.E.; Manley-Harris, M. Anal Bioanal Chem 2013, 405, 5311–5319.
- Gill, B.D.; Indyk, H.E.; Woollard, D.C. J AOAC Int 2016, 99, 30–41.
- Gill, B.D.; Indyk, H.E.; Kobayashi, T.; McGrail, I.J.; Woollard, D.C. J AOAC Int 2020, 103, 1293–1300.
- Indyk, H.E.; Saldo, S.C.; White, P.M.; Dole, M.N.; Gill, B.D.; Woollard, D.C. Int Dairy J 2016, 56, 33–37.
- Auer, F.; Jarvas, G.; Guttman, A. J Chrom B 2021, 1162, 122497.
- van Leeuwen, S.S. Nutrients 2019, 11, 2684.
- Contarini, G.; Povolo, M. Int J Mol Sci 2013, 14, 2808–2831.
- Cossignani, L.; Pollini, L.; Blasi, F. J Dairy Sci 2019, 102, 5871–5882.