“The shellfish of my home may be small, but they nourish the people”
This whakataukī speaks of the value of kaimoana shellfish to the traditional Māori diet: small, yet mighty. Their nutritional value, sought-after taste and ready accessibility to Aotearoa - New Zealand’s earliest settlers made them an essential part of the traditional coastal Māori diet and many, including pāua, were considered taonga (treasures). Kaimoana was also a valued trading commodity for coastal peoples, exchanged with inland tribes in return for forest products such as birds and rats.
Today, kaimoana shellfish are still highly prized for their nutritional value and are regularly consumed, though more commonly as a product of farming than of foraging. New Zealand’s Greenshell™ mussel and Bluff oyster industries are now worth more than 30 million NZD and are claimed to be some of the most sustainable aqua culture practices in the world.2
But, as chemists, why should we be interested in these squishy little species? Worldwide, shellfish are renowned as an excellent source of lipids, including omega-3 polyunsaturated fatty acids (PUFAs). Shellfish are also a particularly good source of phospholipids and the combination of omega-3 PUFAs within phospholipid structures makes for a highly diverse class of molecules with potential biological functions. This diversity, along with their biological applications, makes these an interesting and rewarding class of molecules to study.
Overseas research has characterised the phospholipid composition of a range of shellfish species: Mediterranean mussels, blue mussels, oysters, clams and whelks.3 Three major phospholipid classes are most commonly reported: phosphocholines, phosphoethanolamines and ceramide aminoethylphosphonate, alongside many other minor phospholipid classes,3 some of which are depicted in Fig. 1.
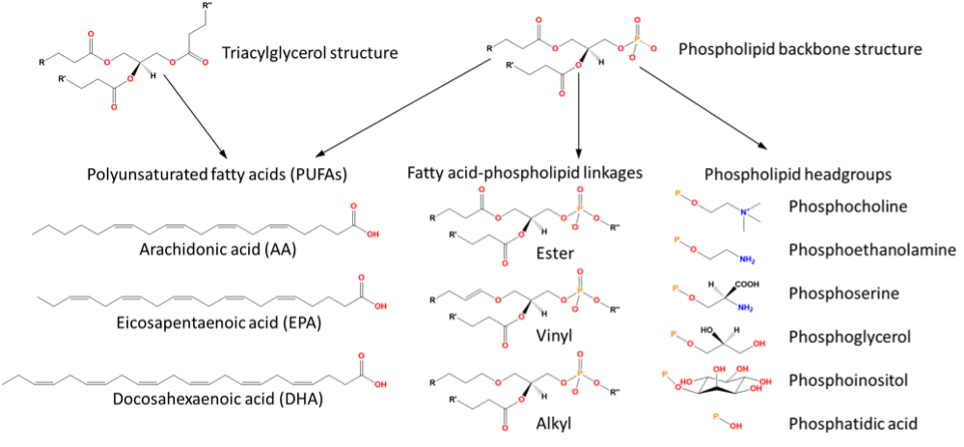
However, New Zealand lipid research on kaimoana shellfish has been confined almost entirely to the commercially relevant Greenshell™ mussel and the lipid profiling techniques have focused primarily on fatty acid analysis by gas chromatography-mass spectrometry (GCMS) or lipid class analysis by thin layer chromatography (TLC) and 31P nuclear magnetic resonance spectroscopy (31P NMR).4 But beyond Greenshell™ mussels are many other kaimoana shellfish with unexplored lipid chemistry, some of which are shown in Fig. 2.
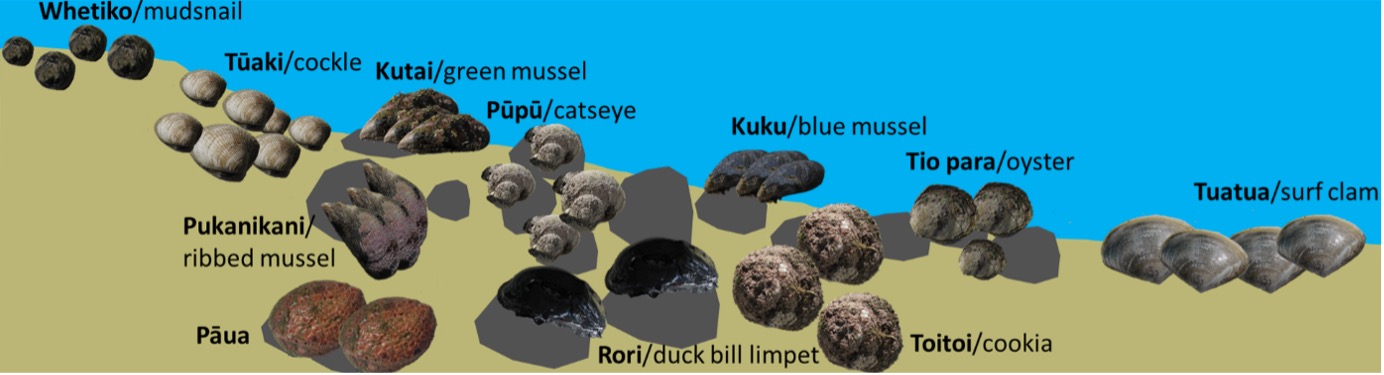
Of course, we are not the first to learn about these species. Many of these species have a strong association with Mātauranga Māori, relating to the time of year that they should be eaten, their health benefits and even how to translocate them in an early form of aquaculture.5 Sustainability was always key to the traditional collection of these kaimoana, and is all the more important today. This research aims primarily to add value to our taonga species by increasing our understanding and appreciation of the world around us and promoting sustainable use to provide benefit to all.
What is a phospholipid?
Lipids are a class of molecules that include all the things we’d recognise as fats and oils, such as cooking oil, wax or adipose tissue. But they also include a few things that might not jump immediately to mind, for example forming a key part of cell membranes or acting as signalling molecules in our bodies.
Fatty acids form one of the major lipid classes and are incorporated into many more complex lipids. These complex lipids can be broadly grouped into ‘neutral’ and ‘polar’classes. ‘Neutral’ lipids include tri-, di- and mono-acylglycerols, which consist of fatty acids esterified to a glycerol backbone (Fig. 1). These are primarily used for energy storage in marine organisms and are the easiest lipids to extract and purify, so they have become the lipid class commonly found in supermarket omega-3 supplements. ‘Polar’ lipids, on the other hand, are much more complex lipid classes, and the most common of these are phospholipids. These consist of one or two fatty acids attached via ester, alkyl, or vinyl bonds at the sn-1 and/or sn-2 positions of a glycerol backbone, with a phosphate or phosphonate group at the sn-3 position. To the phosphate is then attached an organic headgroup, which can vary in size from a small choline group right up to an entire other phosphate-glycerol-fatty acid group. There are five common classes: phosphocholine; phosphoethanolamine; phosphoserine; phosphoinositol and phosphoglycerol, their precursor being phosphatidic acid (Fig. 1).6 Lyso- variants of each class and subclass can also be formed from selective hydrolysis of fatty acids by phospholipases.
The lipid structure can be further modified by the addition of other structures such as ceramides, which form the phosphonolipid class. Phosphonolipids can be formed by the attachment of 2-aminoethylphosphonoic acid (ciliatine) to either a ceramide, diacylglycerol or carbohydrate glycolipid type. The phosphonolipid ‘ceramide 2-aminoethylphosphonate’ is often found in marine organisms. These lipids are part of the sphingolipid class, which contain a long chain sphingoid base backbone and are characterised by a carbon-phosphorous bond.
In combination, these modifications to the phospholipid structure create a lipid class with thousands of potential molecular structures.6 Characterising the phospholipid composition of kaimoana shellfish is a satisfying challenge to tackle, and most lipidomics studies identify 150–250 unique phospholipid molecules.3 This requires a strategic approach. We can use the somewhat regular structure of the molecular class to our advantage and usea raft of analytical techniques to build up a picture of the phospholipid profile of any one species.
The history of lipid analysis started with gas chromatography (GC) to identify different fatty acids, before TLC and high performance liquid chromatography (HPLC) techniques were developed to identify different lipid classes. 31P NMR also provides insight into the range of phospholipid classes present. More recently, mass spectrometry (MS) techniques have been developed to facilitatethe study of both fatty acids and lipid classes simultaneously using fragmentation patterns to identify the molecular structures. Today, liquid chromatography coupled to mass spectrometry (LCMS) is considered the gold standard for phospholipid analyses,3 and a common workflow is shown in Fig. 3.
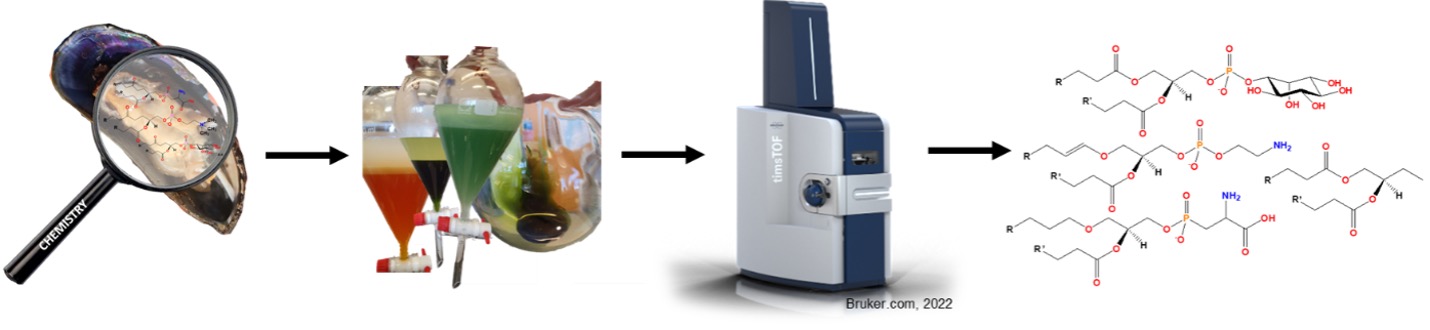
These analytical techniques work additively, so carefully combined approaches allow us to understand the lipid profile of kaimoana species.
Phospholipids for improved omega-3 bioavailability
The potential variation present within the class of phospholipids can give a highly tuneable molecule, with the potential to perform multiple roles in an organism and be affected by many environmental factors. For example, marine creatures in cold environments tend to accumulate more PUFAs, which help maintain better membrane fluidity at low temperatures. In our bodies, it is well established that omega-3 PUFAs are the key lipid structures that confer health benefits and are a common supplement, readily available on supermarket shelves. These are biosynthesised by marine algae and bioaccumulated in filter-feeders and grazers.7
Two omega-3 PUFAs are essential: eicosapentaenoic acid (EPA) and docosahexaenoic acid (DHA) (structures shown in Fig. 1). EPA is a key prostaglandin precursor, while DHA is the major lipid component of the brain and nervous tissue, and deficiencies in either of these molecules is a sign of multiple neurodegenerative diseases. Our bodies do not do a good job of biosynthesising these long-chain omega-3polyunsaturated acids, with only a tiny contribution coming from conversion of the primarily plant-sourced α-linolenic acid.8 Furthermore, the process of moving DHA from the gut to the brain is complex, with many biological hurdles for the molecule to overcome. For example, only free fatty acids and lyso-phospholipids appear able to cross the highly selective blood-brain-barrier.8 Therefore, it is ideal to include the most direct sources of essential omega-3 PUFAs like EPA and DHA in our diet.
Fish have long provided the major source of our omega-3 PUFAs, and I expect many of us are familiar with the dietary recommendation to include oily fish in our diet at least once a week.9 However, shellfish also provide an excellent source of omega-3 PUFAs and their production, when done well, can be more sustainable than fishing. Shellfish are also rich in phospholipids, and the combination of phospholipids and omega-3 PUFAs has been the topic of interesting research in recent years. Specific esterification of omega-3 PUFAs to different phospholipid structures has been shown to modify the bioavailability of these omega-3 PUFAs.8,10 A more bioavailable source of omega-3 PUFAs means that our bodies can more efficiently use what we consume and it may be possible that adequate omega-3 PUFA intake, particularly DHA, can help maintain our brain’s health into later years.8
When comparing the relative bioavailability of omega-3 PUFAs in different lipid forms, conventional triacylglycerol and ethyl ester omega-3 supplements score the lowest. Free fatty acids are more bioavailable, and phospholipids are the most readily absorbed form. However, bioavailability also varies between phospholipids, and phosphocholines and phosphoethanolamines provide the best omega-3 PUFA bioavailability.10 These are two of the most common phospholipid classes found in shellfish, which is very promising for the potential efficacy of these species as foods with the potential application to help improve brain health.3
And where might we find these omega-3 PUFA phospholipids, I hear you ask. Do they have to be synthesised via costly, complex organic protocols? Are they difficult to extract, or to prepare? Well, it doesn’t seem so to me. Kaimoana shellfish may provide the answer: naturally rich in omega-3 fatty acids, including DHA and EPA, along with a diverse phospholipid composition, they may just be a perfect source of these brain-sustaining molecules. “Ahakoa iti te pipi o tōkukāinga, he waiū tangata tonu,” these species are indeed small, yet mighty. It is important to remember that many of these species are unique to Aotearoa - New Zealand and as taonga species, any and all applications should be done in a sustainable manner, with appropriate collaboration and engagement with local communities and with Mātauranga Māori.
Acknowledgements
This project is carried out in consultation with Kāti Huirapa Rūnaka ki Puketeraki and is funded by a University of Otago doctoral scholarship and supported by Plant & Food Research Limited, New Zealand. My thanks go to all involved for their support and advice.
References
1. William, H.W. He Whakataukī, he Titotito, he Pēpeha; Gisborne, Te Rau Kahikatea, 1908.
2. Aquaculture New Zealand. Aquaculturefor New Zealand, a Sector Overview with Key Facts and Statistics for 2020. 2020.
3. Laudicella, V. A.; Whitfield, P. D.; Carboni, S.; Doherty, M. K.; Hughes, A. D. Rev. Aquac. 2020, 12,678–702.
4. Miller, M. R.; Pearce, L.; Bettjeman, B. I. Nutrients 2014, 6, 1454–1474.
5. Wehi, P.; Cox, M.; Roa, T.; Whaanga, H.J. Mar. Isl. Cult. 2013, 2, 59–68.
6. Sud, M.; Fahy, E.; Cotter, D.; Brown, A.; Dennis, E. A.; Glass, C. K.; Merrill, A. H., Jr; Murphy, R. C.; Raetz, C.R. H.; Russell, D. W.; Subramaniam, S. Nucleic Acids Res. 2007, 35 (Suppl 1), D527–D532.
7. Zhukova, N. V. Biomolecules 2019,9, 857.
8. Lacombe, R. S.; Chouinard-Watkins, R.;Bazinet, R. P. Mol. Aspects Med. 2018, 64, 109–134.
9. NHS. Fish and Shellfish. Eat well, 2018. https://www.nhs.uk/live-well/eat-well/food-types/fish-and-shellfish-nutrition/(accessed 15/10/2022).
10. Chouinard‐Watkins, R.; Lacombe, R. S.; Metherel, A. H.; Masoodi, M.; Bazinet, R. P. Mol. Nutr. Food Res. 2019, 63, 1801224.