Abstract
The role complexity can play in understanding societal changes (including war), discovery and innovation (including in science and technology), as well as in biology has become increasingly recognised. However, aspects of complexity’s similarity to self-organisation appears to enable its extension to chemical processes and physics, and thereby may have the potential to redefine what constitutes life.
Graphical abstract

Introduction
The bibliography in Eric Scerri’s most recent history of the development of the periodic table of the chemical elements provides a comprehensive list of ‘descriptive’ narratives, which include much of what practising scientists would describe as their ‘scientific literature’.1 Each such narrative typically incorporate an account of scientists’ experiment(s), comparison with previous investigations, inferences and conclusions drawn from the experiments and – often – the opportunities for future research. In many of these narratives the chemical elements are effectively the characters in the stories, supported by a cast of discoverers, chemists, and physicists. Another example of such a narrative by Scerri is that in which he describes “the discoveries [of seven particular elements] involved intricate stories of epic labours, inspired detective work, scientific passions, collaborations, competitions, and hopes repeatedly raised and dashed.”2 In another example, Scerri turns the tables somewhat on this element-focused approach, focusing on “what he [Scerri] terms the ‘little people’ who although ‘largely forgotten today yet in their time made pivotal contributions to our knowledge of how electrons are distributed around the nucleus of an atom’.”3
Fig. 1 shows the five-year moving average plot of the variation with time of the number of such narratives published since 1869 (the year in which Mendeleev’s periodic table was published) and recorded in Scerri’s history.1 The number of publications varies with time, showing several maxima and minima.
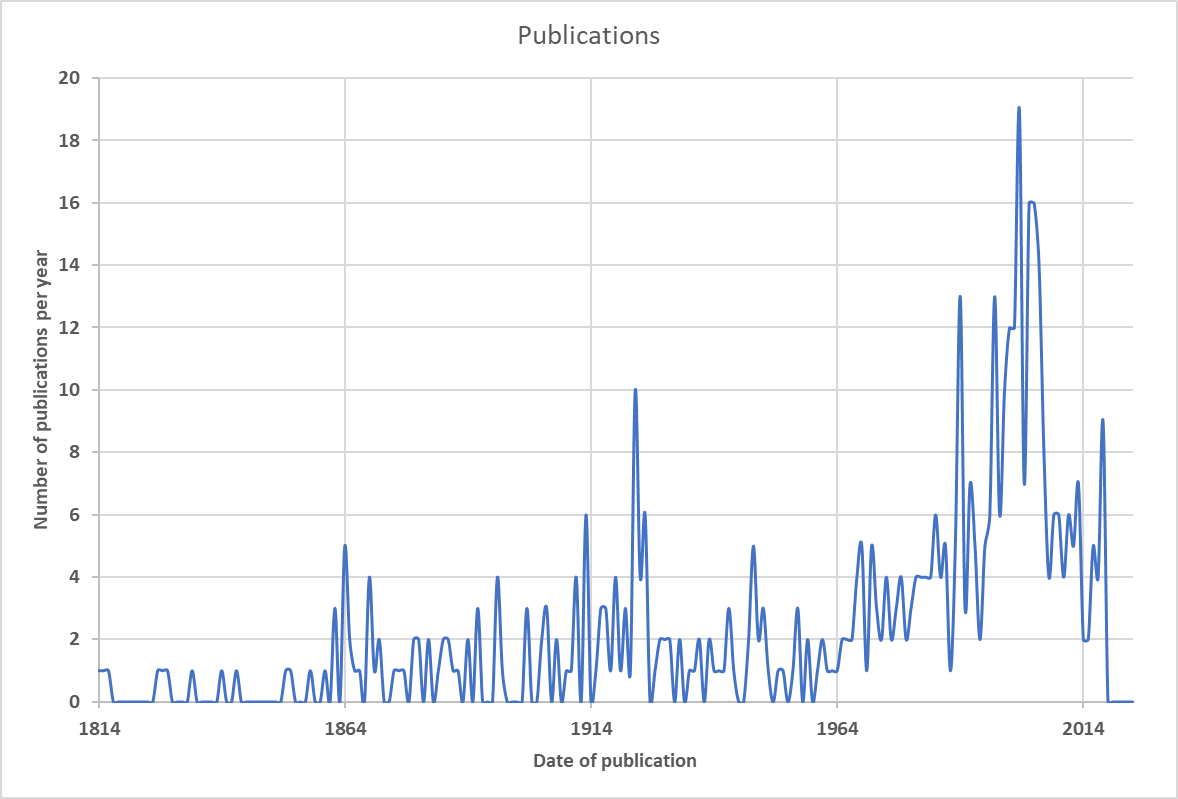
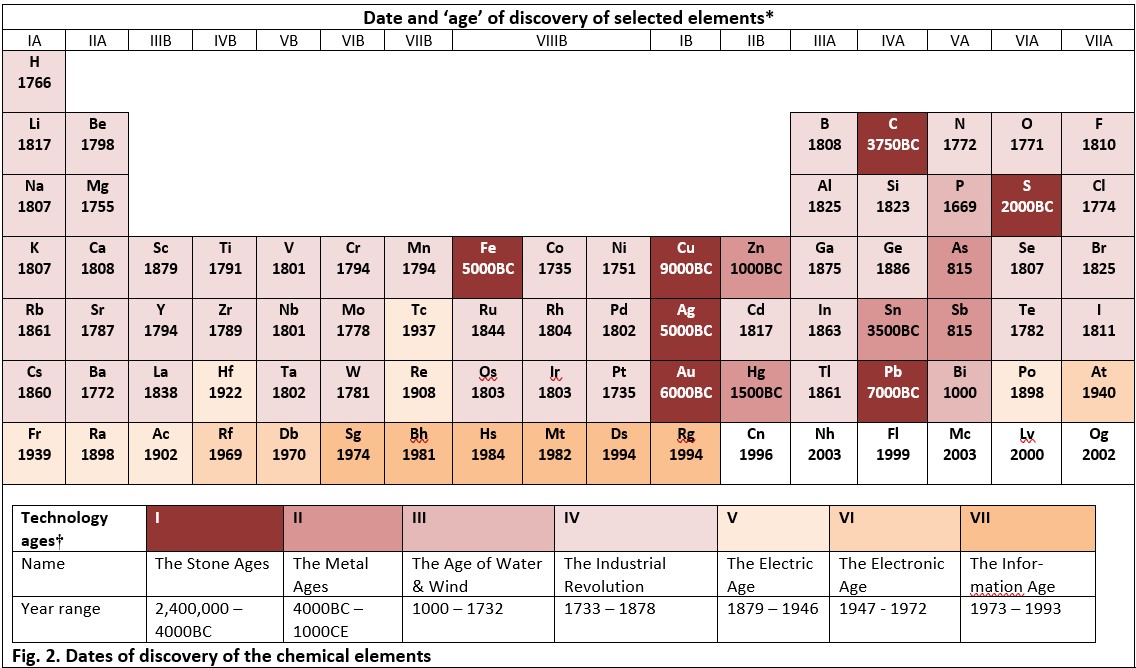

The dates of discoveries of the chemical elements (Fig. 2) are plotted in Fig. 3,4 and show a similar variation with time to Fig. 1. This suggests – but does not necessarily prove – that publications related to the periodic table may have stimulated some of the investigative work that led to the discovery of further chemical elements, and/or the reverse: that discovery of elements may have prompted further research. In other words, the trends of Fig. 3 might be attributed to a complex but mutual feedback of research and discovery.

These variations of publications and element discovery with time have a similarity in form to the natural self-organising systems recently discussed by Golledge:
“Everything in the world we see today is there because of processes that over four-and-a-half billion years have gradually refined the ways simple building blocks are put together. This process of assembly has given rise to complex structures that exhibit complex behaviours, even though the laws of physics dictate that it should be chaos, rather than order, that increases over time……processes of self-organisation enable that complexity, and also the characteristic patterns of behaviour that many natural systems share – from plate tectonics to ecological diversity, from the global climate to the beating of our hearts.”5
There is an obvious linkage between elements’ discovery (Fig. 3)4 and publications about them (Fig. 1): the former generally proceed the latter. However, once the periodic table was being developed, the existence of some elements was predicted before they were actually discovered. Fig. 4 shows the variation of the combined number of discoveries and publications per year. This is a ‘sawtooth’ pattern, with the combined number of discoveries and publications attaining irregularly timed maxima, prior to marked decreases and subsequent increases. As is shown later in this paper, this pattern resembles those in Fig. 5, which are attributed to ‘complexity’.

Complexity in social systems
Complexity is not a new idea: it was used by scholars to explain the collapse of civilisations,6 and was popularised by Diamond in his book How Societies Choose to Fail or Succeed.7 In support of this stance, Cline asserted that civilization “contains a collection of many interacting objects or agents”.8 He notes that in one aspect of complexity theory, behaviour is affected by their memories and feedback from what has happened in the past. People are able to adapt their strategies, partly on the basis of their knowledge of previous history. He also states that the system is typically “alive”, meaning that it evolves in a non-trivial and often complicated way, one that is also “open”, meaning that it can be influenced by its environment. Another scholar posits:
“as such systems become more complex, and the degree of interdependence between their constituents grows, keeping the overall system stable becomes more difficult”, ‘hyper-coherence’ occurring “when each part of the system becomes so dependent upon each other that change in any part produces instability of the system as a whole.”9
This concept has been applied to the world’s empires – both historic and recent, highlighting the differences between them, and noting that there is not a single model that explains their formation and demise.10 Complexity in processes thus can produce a range of outcomes, which may not necessarily have been predicted – or, indeed, predictable. A representation of the range of possibilities is given in Table 1.11

While one of these types of exchange could ‘drive’ a historic incident or period of change, more than one exchange might be involved, and, moreover, exchanges are not required to be in a specific order. Such is the nature of complexity.
As a specific example, Woolf cites a group of states in Roman times - so-called ‘peer polities’ – “which for a while seem to develop on parallel lines at an accelerated rate as they compete with each other and learn from each other’s successful experiments and mistakes”.12 He goes on to observe, “Peer-polity systems have other effects, however, including a certain amount of institutional inbreeding and a tendency to limit the success of their members.” A consequence of this change of focus during a time of peer polities for fourth-century Greece resulted in “successive leading city states [being] brought down to size by alliances made among the others”; a similar effect is described as having occurred in sixth-century Etruscan cities.
While the examples above apply complexity to the demise of systems, it is also suggested as being applicable to societal advancement: the Neolithic building of stone circles has been suggested as an example:
“Neolithic groups [across the British Isles] proclaimed to the world that they valued their future and chose to shape it to their advantage. Because each structure brought order to the natural world, set in stone the cyclic changes that watermarked their daily lives and that repeated dependably from year to year, one generation to the next. Predicting the ebb and flow of seasonal changes, the circles these settlers constructed became the basis of a contract with their environment. With the help of majestic stone markers, they could each year be better prepared, more prosperous and prolific than the year gone by. The short-lived struggle of construction locked in long-lasting benefits and adaptations that eased their toil and enabled growth.”13
If this idea is applicable to other social processes, it suggests that, for example, the development of scientific theories can also proceed via a variety of means. Specifically, while some innovative theories might be adopted after a short period of contention, in essence, Kuhn’s idea of a revolutionary episode of scientific discovery interspersed between episodes of so-called ‘normal science’,14 others may take longer, such as Scerri’s view that “science progress is far more organic than usually supposed,”15 i.e. it is evolutionary rather than revolutionary.16
Seeking universality in complexity, Luczak-Roesch noted:
“Whether it’s the movement of wildfires or antibiotic resistance in bacteria, the genesis of successful start-ups or the flourishing of social movements on the internet, emergence [italics added] is observable everywhere in the systems that surround us…” defining emergence as “the spontaneous and unexpected interactions between parts of very large and complex systems that lead to changes in the entire system. It’s the chaotic events in the order we strive to apply to the world.”17
More pragmatically perhaps, Nussbaum and Sen “believe society should enlarge and enrich people’s capabilities, most of all their capacity to co-operate; [noting that] modern society instead diminishes it”.18 If this is valid, perhaps a lack of co-operation is an element of complexity and a contributing cause of some historical crises.
Complexity in living systems
Haskell, a biologist who is utterly accepting that plants and animals communicate with each other for mutual benefit sees no necessity to either anthropomorphise or otherwise justify it.19 More recently, Schlanger, wary of previous shoddy but widely reported experiments in the 1970s into so-called “plant intelligence”, is reported by the reviewer (the same Haskell as above) of her recent book as being left in no doubt that:
“…conscious or not, plants sense their surroundings and make sophisticated decisions..….In an age when we often feel alienated from a living world in crisis, it is good to be reminded that other species have agency and acumen. Plants have thrived on Earth for half a billion years. They embody not only intelligence but wisdom about how to flourish in the face of change.”20
A few years earlier, it was suggested that “discoveries about the complicated and proactive sensory systems of plants dignify them out of the passive role of ‘service providers’… moving them to a more existential level.” However, the same ‘dignity’ was not extended to entities not generally recognised as living:
“But this is all a parallel universe. Just as quantum physics and string theory have no relation to our everyday experience of the material world, so the discoveries of the new botany don’t impact on how we relate to living plants beyond validating them as autonomous beings.”21
Complexity in apparently non-living systems
As mentioned earlier in this paper, the variations of publications and element discovery with time referred to earlier in this paper have a similarity in form to the natural self-organising systems discussed by Golledge.5 These include the episodic (but non-cyclic) variation that are involved in the timescale of extinctions of life on earth,22 the coming together and dispersal of tectonic plates,23 the rise and fall of sea-level,24 and the variation in the polarity of the Earth’s magnetic field,25 i.e. the variation with time of ‘geomagnetic reversals’, as shown in Fig. 5.26
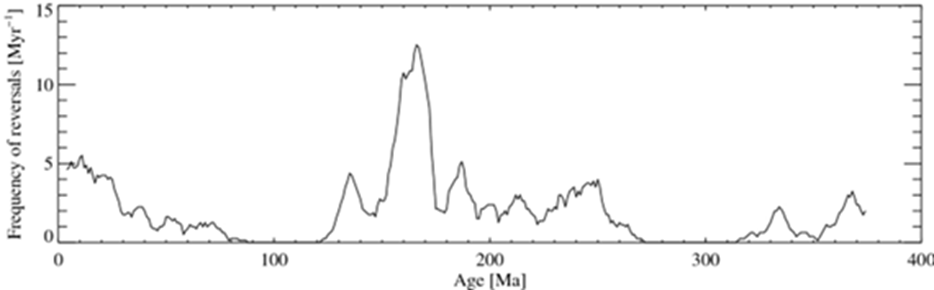
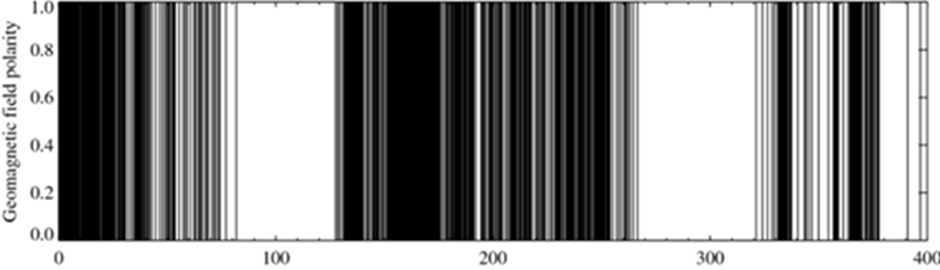
Fig. 5. An example of self-organisation behaviour. Upper: frequency of geomagnetic reversals, as number of reversals per million years.26 Lower: the black and white vertical lines and bands correspond to the times when the geomagnetic field is of ‘normal’ or ‘reverse’ polarity, measurable in basalts of mid-oceanic ridges.26
Further examples of these types of plots are also shown in Fig. 6.
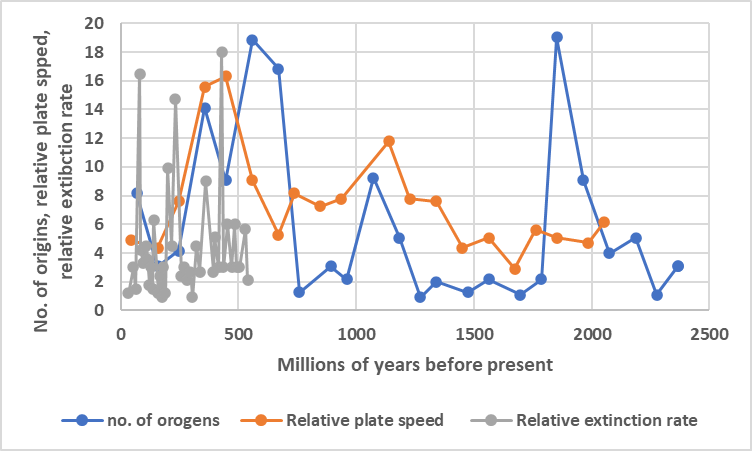

Fig. 6. Typical ‘saw-tooth’ and ‘two-slope’ plots related to plate tectonics, climate change, paleomagnetism and life on earth. Upper: re-drawn plots of number of orogens (i.e. continental collisions),28 relative plate speed28 and relative extinction rates.29 The plots related to orogens and plate speed show some similarity, and so may be associated. Lower: redrawn plots of global mean temperatures over the last 500,000 years (0.5Ma),30 and the approximate minimum and maximum intensity of the paleomagnetic field of the earth over the same period.31 There is a weak similarity between the overall trends of paleomagnetic intensity and paleotemperatures with time, indicating a possible relationship between these parameters.
Such trends have been described as a consequence of ‘nature’s invisible hand’ at work,27 envisaging them occurring as the consequence of a combination of memory, irreversibility, and assembly.5 A quarter of a century earlier, this type of process was interpreted as a ‘criticality’: “a minute perturbation to one part of the system that can shift – or even crash – the entire system.”32
The maxima (or minima) in Fig. 5 are neither the same size (shown as ‘frequency of reversals’), nor separated by a constant interval of time. This suggests that there is unlikely to be a single or simple explanation for the curve overall. This recognition is reasonably recent: such changes with time as those shown in Fig. 5 and Fig. 6 would once have been attributed to relatively simple external influences. One such example is the change in mineralogy that occurs as slabs of oceanic crust are subducted into the earth’s mantle. As the pressure and temperature changes, minerals that were originally stable become less stable and may be converted into others. At one time it was thought that such mineralogical transformations in this metastable pressure-temperature tectonic regime might occur sufficiently rapidly to generate shock waves, i.e., earthquakes.33 Another example is the explanation for dramatic crystallisation of ice in places such as the Sea of Okhotsk,19,34 a rather earlier example of which was described by Welzl (1932) as:
“An icy pulp was forming, the chunks of ice were growing, getting wider, the pulp was up to a foot thick, it was getting clotted, chunks of ice shifted closer and closer, until they formed large irregular bodies of pulp – and then suddenly, almost at the same second – bang! It all froze and the icy covering was whisked across the sea farther than the eye could reach.”35
The ‘bang!’ in Welzl’s text suggests that this freezing occurred with the output of a shock wave, possibly caused by cavitation – the collapse of bubbles in supercooled water that was proposed in 1976,36 and subsequently supported in 2020.37 Alternative explanations, attribute the freezing to variations in the salt content of the developing ice-sheet,38 or the combination of “water containment, a means to bring down the water temperature and a source of turbulence.”39
On a much smaller scale Chubynski et al. have described the ‘tailoring’ of the collision of drops, noting “that for a droplet to bounce the speed of collision must be just right. Too fast, and the momentum of the drop flattens the air cushion too thinly. Too slow, and it gives the van der Waals attraction just time to take hold.”40 This ‘tailoring’ is reminiscent of the observation:
“[From] experiments [that] provide a strong evidence for the many-body nature of vdW [van der Waals] interactions in complex materials, it is expected that they are just the ‘tip of the iceberg’ in the ocean of molecules and materials. The complex interplay of size, dimensionality, and polarisation response will continue to surprise us and lead to new insights into vdW interactions, and ultimately to better control over the self-assembly [italics added for emphasis] of complex polarizable molecules and materials into useful ensembles.”41
More generally, it has been suggested that there is a range of possibilities for behavioural variation as a consequence of self-organisation in geological materials:
“In some cases, several interactions compete, often on different time and distance scales to determine a mineral’s properties. Thus, the resulting structure is often quite finely balanced, and small perturbations in external parameters can thus cause significant changes in properties. In this sense, minerals have the degree of complexity needed to be ‘interesting’ or ‘smart’[italics added for emphasis] or ‘compliant’, to have properties tailored for very specific applications.”42
In fact, the recognition of ‘smartness’ and ‘self-assembly’ in geochemistry are not that new, with symmetry-breaking being considered to be an important aspect of the process in 2006,43 and a fractal-based ‘discovery’ advocating in 2011:
“… energy/matter flowing through and comprising the Universe evolves as a multiscale, self-similar structure-process, i.e., as a self-organizing fractal. This means that certain organizational structures and processes are scale-invariant and are reproduced at all levels of the organizational hierarchy. Being a form of symmetry, scale-invariance naturally lends itself to a new discovery method that allows for the deduction of missing information by comparing scale-invariant organizational patterns across different levels of the organizational hierarchy.”44
Subsequently, a geochemical perspective45 was highlighted in a recent conference paper:
“For a long time, this topic [the self-organisation concept for geological and ore-forming processes] has been examined from a thermochemical-mechanical perspective, seldom along with the numerical modelling of the processes. We proposed applying the concept of self-organisation to mineral deposits and geological processes in general. Recognising geological systems, mineral deposits and ore bodies as ordered structures implies to consider them as a system in which their components and properties are distributed in space and time, providing fundamental aspects of understanding them…”46
Although that paper continues by saying, “Thus, the complexity in describing connections and feedback between contributing key processes can be reduced, leading to a better understanding and achievable numerical simulations,” it may ultimately prove preferable to freely acknowledge complexity as the cause of the temporal variation in plots such as Figs. 3 and 4, rather than to seek to limit it. Equally significant may be the suggestion that links self-organisation to the development of life itself.47
A contentious extension
Rather more contentiously, such observations might open the door to a reconsideration of ‘resistentialism’, a term coined by humourist Paul Jennings to explain seemingly spiteful behavior manifested by inanimate objects.48 ‘Resistentialism’ was subsequently defined as “seemingly spiteful behaviour manifested by inanimate objects”).49 Now included in the Oxford English Dictionary, it is there defined as “a mock philosophy maintaining that inanimate objects are hostile to humans or seek to thwart human endeavours”. Jennings cited phenomena such as the determination of keys to hide from their owner and the elusiveness of bouncy balls as examples of resistentialiam, which has been summarised in the four words: ‘les choses sont contre nous’, translated as: ‘Things are against us.’50 The contrary behaviour of the inanimate has been updated for the 21st century.51 Such observations might even invite a revisiting of the generally discredited late-nineteenth-century concept of ‘vitalism’,52 although some might consider this a step too far.
Conclusions
It will be interesting to see whether future scholars agree, not only with Mabey’s hope that “we can respectfully maintain the independence of our kingdoms [i.e. plant and animal], not as irrevocably separate orders, but as two linked pathways to the challenges of being alive,” but also with his assertion that the behaviours that characterise quantum physics are confined only to the submicroscopic realm.”21 However, this may not be the case: the selection of research ‘tales’ in this paper raise the question of the nature of life itself. In essence, is life is only restricted to biology, and, therefore, other processes are merely ‘like-life’, or does the definition of life needs amending to include the agents of self-organisation.
Notes and References
- Scerri, E. The Periodic Table: Its Story and its Significance, Oxford University Press: New York, 2nd ed., 2020, 421-428
- Sacks, O. In: Scerri, E. A Tale of Seven Elements. Oxford University Press, New York, 2013, ix-x
- Atkins, P. In Scerri, E. A Tale of Seven Scientists and a New Philosophy of Science. Oxford University Press, New York, 2016, ix-x
- In this diagram (Fig. 2) the dates of discovery of the chemical elements are from Periodic table by era of discovery, https://www.periodictable.one/history/periodic-table; the technology ages from Bunch, B.; Hellemans, A. (eds). The Timetables of Technology. A Chronology of the Most Important Events and People in the History of Technology. Simon and Schuster: New York, 1993
- Golledge, N. Earth, Life, and Climate –In Search of Nature’s Invisible Hand. Inaugural lecture, Victoria University of Wellington, 2023. https://www.youtube.com/watch?v=tpENv8ge41M; see also Golledge, N. R. Feedback: Uncovering the Hidden Connections Between Life and the Universe. Rowman and Littlefield: Lanham, Maryland (United States), 2023
- Johnson, N. Simply Complexity. A Clear Guide to Complexity Theory. One World: Oxford, 2007; Darwin, J. After Tamerlane: The Rise and Fall of Global Empires, 1400 – 2000. Penguin: London, 2008
- Diamond, J. Collapse: How Societies Choose to Fail or Succeed. Viking: New York, 2005
- Cline, E.H. 1177 B.C.: The Year Civilization Collapsed. Princeton University Press: Princeton and Oxford, 2014
- Dark, K.R. Waves of Time: Long Term Change and International Relations. Continuum: New York, 2001
- Roberts, A. Superstates: Empires of the 21st Century. Polity, 2022 [Cited in Stevenson, T. London Review of Books, 2022, May 4, 11-12]
- Sennett, R. Together: The Rituals, Pleasures and Politics of Cooperation. Yale University Press: New Haven, 2012, 72-86
- Woolf, G. Rome: An Empire’s Story (2nd edition). Oxford University Press: Oxford, 2022, 41-42
- Golledge, N. Spinoff 2024, July 5. https://thespinoff.co.nz/books/05-07-2024/a-contract-with-their-environment-explaining-the-incredible-power-of-feedbacks
- Kuhn, T. The Structure of Scientific Revolutions. University of Chicago Press: Chicago, 1962
- Scerri, E. A Tale of Seven Scientists and a New Philosophy of Science. Oxford University Press: New York, 2016, 196
- Hodder, P. Foundations of Chemistry 2018, 20, 99-110
- Luczak-Roesch, M. Newsroom 2020, January 12
- Nussbaum, M; Sen, A. The Quality of Life. Clarendon Press: Oxford, 1993. Cited in Sennett, R. Together: the Rituals, Pleasures and Politics of Cooperation. Yale University Press: New Haven and Londin, 2012
- Haskell, D.G. Sounds Wild and Broken: Some Marvels, Evolutionary Creativity and the Crisis of Sensory Extinction. Black Inc.: Collingwood (Australia), 2022
- Schlanger, Z. The Light Eaters: How the Unseen World of Plant Intelligence Offers a New Understanding of Life on Earth. Harper, 2024. Cited in: Haskell, D.G. Scientific American 2024, 330 (5), 88
- Mabey, R. The Cabaret of Plants. Profile: London, 2015, 338
- Van Andel, T.H. New Views on an Old Planet, 2nd edition. Cambridge University Press: Cambridge, 1994, 371-376, fig. 19.1
- Nield, T. Supercontinent: Ten Billion Years in the Life of Our Planet. Granta Books: London, 2007; Van Andel, T.H. New Views on an Old Planet, 2nd edition. Cambridge University Press: Cambridge, 1994, 376-382, fig. 19.4
- Mortimer, N.; Campbell, H. Zealandia: Our Continent Revealed. Penguin: London, 2014
- Van Andel, T.H. New Views on an Old Planet, 2nd edition. Cambridge University Press: Cambridge, 1994, p. 37, fig. 2.5
- Carbone, V.; Alberti, T.; Lepreti, F.; Vecchio, A. Scientific Reports 2020, 10, article no. 13008
- The term ‘invisible hand’ derives from economics: “The invisible hand is a metaphor for the unseen forces that move the free market economy. Through individual self-interest and freedom of production and consumption, the best interest of society, as a whole, are fulfilled.” https://www.investopedia.com/terms/i/invisiblehand.asp#:~:text=The%20invisible%20hand%20is%20a,as%20a%20whole%2C%20are%20fulfilled The assertion that: “… economics is the only discipline, science, or field of enquiry where practitioners pride themselves on having something invisible as the foundational concept of their discipline”, as in Basu, K: Beyond the Invisible Hand: Groundwork for a New Economics. Princeton University Press: Princeton, 2010, would presumably be refuted by Golledge.
- Condie, K.C. Geoscience Frontiers 2018, 9 (1), 51-60, https://doi.org/10.1016/j.gsf.2016.09.001
- Sutter, J.D.: How to stop the sixth mass extinction, 2016, https://edition.cnn.com/2016/12/12/world/sutter-vanishing-help/index.html
- Adamo, N.; Al-Ansari, N.; Sissakian, V.K. Engineering 2021, 13 (11), 605-630, http://dx.doi.org/10.4236/eng.2021.1311044
- Roberts, A.P. Geophysical Research Letters 2008, 35 (17), https://doi.org/10.1029/2008GL034719
- Bak, P. How Nature Works: The Science of Self-Organised Criticality. Oxford University Press: New York, 1997, 1-3
- Barton, A.F.M., Hodder, A.P.W., & Wilson, A.T. Nature 1971, 234, 293-294 ; Barton, A.F.M.; Hodder, A.P.W.; Wilson, A.T. Chemical Reviews 1973, 73 (2), 127-139
- Iwasaka, S.; Otsuka, J. Frontiers of Marine Science 2021, 8, http://dx.doi.org/10.3389/fmars.2021.713784
- Welzl, J. Thirty Years in the Golden North. Macmillan: London, 1932. Cited in Barton, A.F.M.; Hodder, A.P.W.; Wilson, A.T. Chemical Reviews 1973, 73 (2), 127-139
- Hodder, A.P.W. New Zealand Journal of Geology and Geophysics 1976, 19 (6), 821-826
- Thorne, C.E. Periglacial geomorphology: what, where, when? In Periglacial Geomorphology: Binghampton Geomorphology Symposium, 2, 2020, Chapter 1.
- How does the Arctic Ocean freeze during winters? 2023. https://www.scienceabc.com/nature/the-way-you-freeze-the-arctic-ocean.html
- Barrette, P.D. Cold Regions Science and Technology 2021, 189, https://doi.org/10.1016/j.coldregions.2021.103334
- Chubynski, M.V.; Belousov, K.I.; Lockerby, D.A.; Sprittles, J.E. Physics Review Letters 2020, 124, 084501
- Hermann, J.; diStasio, R.A.; Tkatchenko, A. Chemical Reviews 2017, 117 (6), 4714-4758, https://doi.org/10.1021/acs.chemrev.6b00446
- Navrotsky, A. Complexity and Self-Organization. In Physics and Chemistry of Earth Materials. Cambridge University Press: Cambridge, 1994, 410
- Newth, D.; Finnigan, J. Australian Journal of Chemistry 2006, 59 (12), 841-848
- Kurakin, A. Theoretical Biology and Molecular Modelling 2011, 8 (4), https://doi.org/10.1186/1742-4682-8-4
- L’Heureux, I. Philosophical Transactions of the Royal Society A 2013, 371 (2004)
- Dietrich, S. Goldschmidt Virtual Conference, ORAL-PM-150, 9 July 2021
- Ouazan-Oeboul, V.; Agudo-Canalejo, J.; Gloestanian, R. Nature Communications 2023, 14, article 4496, https://dx.doi.org/10.1038/s41467-023-40241-w
- Jennings, P. The Spectator 1948
- Hellwig, P. Insomniac’s Dictionary, Ivy Books, 1989
- Leslie, I. New Statesman, 2019, 5 June, https://www.newstatesman.com/science-tech/2019/06/gadgets-hate-why-it-seems-your-toaster-conspiring-ruin-your-life
- The Insolence of the Inanimate, 2022, https://glanglish.blogspot.com/2021/11/the-insolence-of-inanimate.html
- Coulter, I.; Snider, P.; Neil, A. Integrative Medicine (Encinatas) 2019, 18 (3), 60-73
- Meyers, C.S. Mind 1900, New series, 9 (34), 218-233