A glowing story: the chemical mechanisms behind bioluminescence in marine organisms
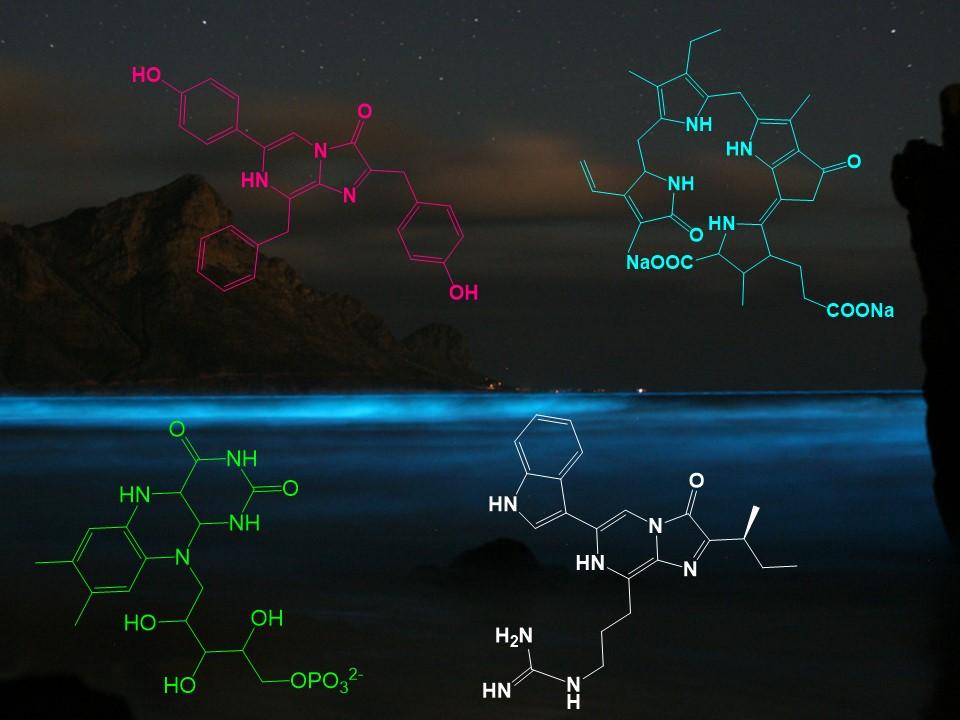
This article introduces bioluminescence in the marine world and examines the chemistry behind this phenomenon in three different marine organisms, namely dinoflagellates, ostracods and jellyfish. It explores how light is produced through specific chemical reactions involving compounds called luciferins, in the presence of luciferase enzymes and driven by oxygen. This article also discusses current and potential applications linked to the understanding of the chemical mechanisms behind bioluminescence.
An introduction to bioluminescence
In the ocean’s depths where darkness is permanent, many marine organisms have the interesting ability to emit visible light to illuminate the surrounding waters, an attribute known as bioluminescence. Bioluminescence is defined as the emission of visible light resulting from a chemical reaction and is estimated to have evolved independently at least 40 times, if not more, across the billion years of life on Earth.1 It serves various ecological functions including predation, defence against predators and reproduction.1-4 Several forms of bioluminescence have been identified, such as bioluminescent glows or sudden flashes, with each serving different functions and purposes.1, 5
Although luminous species can be found among terrestrial organisms (bacteria, insects, worms and fungi), the ability for bioluminescence is still most widespread in marine organisms (Fig. 1).4, 6 At great depths, where light from the surface is entirely absent, more than 90% of organisms exhibit bioluminescence.1 The exact reasons for the contrast in bioluminescence prevalence between marine and terrestrial habitats remains not entirely understood, but factors favouring its evolution in the ocean likely include a long, uninterrupted evolutionary timeline and a significant portion of the habitat existing in perpetual darkness.1
In the marine realm, bioluminescent organisms encompass nearly all taxonomic groups, spanning from bacteria to jellyfish to fish.1 Due to most bioluminescence having evolved in the open ocean, most emission spectra are blue (λmax ~ 475 nm), centred on the wavelength that travels farthest through seawater.1, 5, 7 Green is the next most common colour and is more often observed in species inhabiting benthic zones and shallow coastal waters. This is possibly due to the increased turbidity from particles in the water dispersing blue light and favouring the transmission of longer wavelengths.5 Other bioluminescent colours, such as violet, yellow, orange, and red occur rarely and in most of these cases, their functions and chemistries remain largely unknown.5 Interestingly, unlike ordinary light, bioluminescent light is created through a reaction that efficiently converts chemical energy into visible light without significant loss as heat.2 Surprisingly, the simplicity with which bioluminescence can be observed, especially in terrestrial organisms, contrasts with the complex and fascinating chemical processes responsible for such phenomena.2

Chemical basis of light producing reactions
Over time, the study of bioluminescence has evolved into a collaborative pursuit, involving chemists, biologists, ecologists, and physicists.2 The earliest attempts to understand the chemistry behind these light producing reactions date back to Boyle’s pioneering work in 1667, which demonstrated the essential role of oxygen in all bioluminescence reactions.2 More recently, Shimomura’s groundbreaking research on the jellyfish Aequorea victoria8 and the discovery of the green fluorescent protein (GFP) earned him the 2008 Nobel Prize in Chemistry, shared with M. Chalfie and R. Tsien, marking a significant advance in the understanding of bioluminescent mechanisms.1, 2
At its core, bioluminescence is a type of chemiluminescence reaction driven by enzymes in living organisms.2 The process involves a chemical reaction that generates molecules in excited states, and as these molecules transition back to their ground state, visible light is emitted.2, 9 The starting point of the chemical reaction is a set of compounds called luciferin (from the Latin word “lucifer,” meaning “light–bringer,”). Luciferin’s structure varies between different organisms, but its role remains constant: it reacts with oxygen in a reaction catalysed by an enzyme commonly referred to as luciferase or photoprotein. Photoproteins are enzymes that form stable luciferin complexes and will be explored further in the section “Coelenterazine and the jellyfish bioluminescence”.2
From a chemical perspective, the reaction responsible for bioluminescence can be broken down into key stages resulting in the formation of the oxyluciferin, the light emitter of the reaction (Fig. 2). First, luciferin reacts with oxygen to form an unstable high–energy intermediate, which in all known cases is a peroxide, characterised by an unstable single bond between two oxygen atoms. As this weak oxygen-oxygen bond breaks, it propels the now oxyluciferin into an excited state and as it returns to its ground state, it releases energy in the visible light range, corresponding to the energy gap between these two states.2

In a bioluminescent chemical reaction, luciferins represent one of the primary components and their structures are highly conserved across various phyla.1 Within the ocean, four luciferins, namely dinoflagellate (1), coelenterazine (2), cypridina (3) and bacterial (4), are responsible for the majority of light production (Fig. 3), although there are possibly numerous other light-emitting reactions yet to be discoved.1, 10 Of the four luciferins shown, 2 and 3 are imidazopyrazinones, consisting of a combination of 5- and 6-membered nitrogen-containing rings (Fig. 3).1 1, 2 and 3 appear to be widespread across the oceans, occurring in at least two phyla for 111 and 3,12 and up to nine phyla for 2.1, 5 The explanation for this phylogenetic diversity is not that these organisms are all synthesising the same molecule, but could rather be explained by transmission of luciferin through the food chain.13 In fact, experimental evidence has demonstrated dietary acquisition of luciferin in certain species, such as the teleost fish Porichthys notatus,14 the mysid shrimp Gnathophausia ingens15 and the jellyfish Aequorea victoria.16
Out of the four marine luciferins responsible for most light production (Fig. 3), the detailed mechanisms of action of the three most widespread (1-3) will be explored in the rest of the article.

Dinoflagellate luciferin
Dinoflagellates are an important group of phytoplankton unicellular protists that exhibit a great diversity of both shapes and sizes, and emit blue light around 475 nm.2 The most common and best studied bioluminescent dinoflagellate is Noctiluca scintillans17 (formerly known as Noctiluca militaris) (Fig. 4). Reaching sizes of 1–2 mm, (very large for unicellular organisms which are typically much smaller in size), it has no armour and only one flagellum.2

The dinoflagellate luciferin (1) has been isolated and identified as a tetrapyrrole (Fig. 3), although the exact mechanism of bioluminescence is not completely understood (Fig. 5).1, 9 1 is similar to chlorophyll but differs mainly in the absence of magnesium ions, and the two compounds may in fact be interconverted on a day-night cycle as the cell alternates between photosynthesis and luminescence (Fig. 6).1, 11 The biological function of dinoflagellate bioluminescence has been investigated and it was confirmed that, apart from the immediate deterrence of predators through flashes of light, aposematism (warning coloration) also plays a role.18

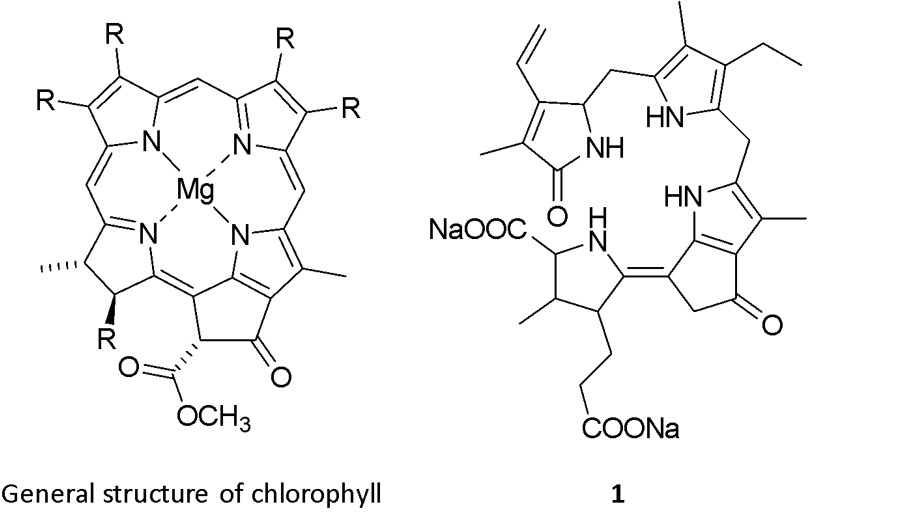
Nowadays, it is even possible to purchase living bioluminescent dinoflagellates. These are usually Pyrocystis fusiformis,19 which are enduring enough to survive transportation for several days. These bioluminescent dinoflagellates have so far been used by aquarists and artists and serve students and teachers in the study of bioluminescence.2
Cypridina luciferin
Ostracods, a type of marine crustacean, are found on the coasts of many oceans as well as occasionally in freshwater. Often referred to as sea-fireflies, they resemble small clams and range in size from 0.1 mm to 2.5 mm.2 Currently, approximately 12,500 living and 20,500 fossil species have been described, however, the number of bioluminescent ostracod species remains unclear.2 Vargula hilgendorfii20 (previously called Cypridina hilgendorfii) stands out as the most well-known bioluminescent member of the class (Fig. 7) and emits light with an intensity maximum of around 460 nm.2
The scientific documentation of sea–fireflies traces back to 1760 but it is highly likely that Vargula hilgendorfii and its bioluminescence have been known in Japan for even longer. In Japanese, this millimetre–sized crustacean is known as umi–hotaru, and mention of its glow, which is triggered by physical stimulation, is widely found in tales and legends. On the northwest coast of Japan’s main island of Honshū, in Toyama Bay, a unique spectacle resulting from the glow of thousands of these organisms can be observed every late spring.2 Remarkably, Japanese soldiers in World War II used to rub dried sea–fireflies in their hands with saliva as a form of emergency lighting.2
The molecular structure of the cypridina luciferin (3) (Fig. 3) began to be examined in Japan shortly after the end of World War II. In the mid-1950s, the later Nobel laureate Osamu Shimomura worked within the group of Yoshimasa Hirata, leading to the purification and isolation of 3,21 making it one of the first marine luciferins to be elucidated.1 Due to the instability of this substrate upon contact with air, isolating the luciferin proved to be challenging.9 In the following years, the bioluminescence mechanism was further investigated and finally verified by Osamu Shimomura in 1971.22 3 is found mainly in cypridinid ostracods, the midshipman fish (Fig. 7), where a dietary link has been found,23 and some other species of fish. Today, it is assumed that ostracods biosynthesise their luciferin from tryptophan, isoleucine and arginine but the details of the pathway are not known.1, 24, 25

According to current scientific understanding, the bioluminescence mechanism of Vargula hilgendorfii can be broken down into several steps (Fig. 8). Initially, luciferase triggers the activation of luciferin by deprotonating 3.2 This enables a single electron transfer with oxygen, leading to the formation of a peroxyanion. Due to its high nucleophilicity, the formation of a 1,2-dioxetanone is favored.2 This is the high energy intermediate (HEI) of the bioluminescence reaction and decays via CO2 elimination, generating the excited state oxyluciferin.2 As the oxyluciferin return to its ground state, it emits light.2
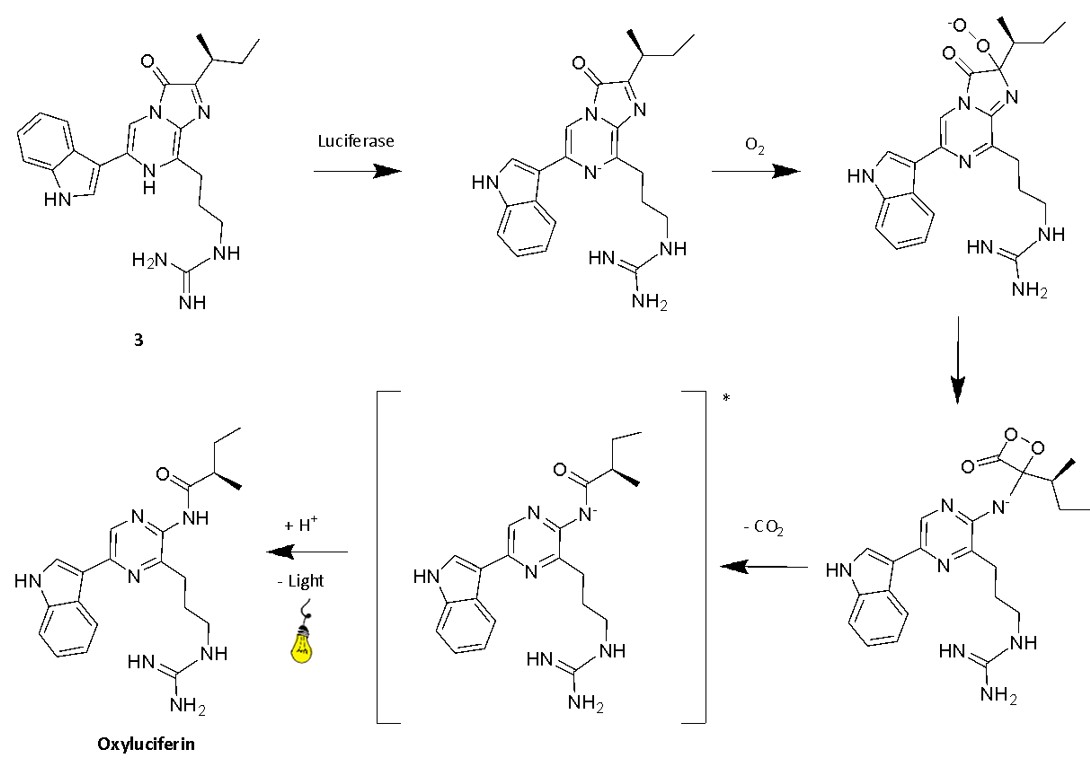
Fig. 8. Mechanism of cypridina bioluminescence, the precise protonation states are still uncertain2
Coelenterazine and jellyfish bioluminescence
The discovery of the bioluminescence process of jellyfish is associated with the work of Osamu Shimomura. His research leading to the discovery of the photoprotein aequorin and the green fluorescent protein (GFP) earned him the 2008 Nobel Prize in Chemistry. The discovery of the GFP started with a rather straightforward observation: many of the bioluminescent jellyfish that have been known for centuries, including Aequorea victoria, naturally emit greenish light. However, when researchers isolated the molecules behind the bioluminescence process and tried to combine them in vitro, they only detected blue light.1 This raised a crucial question: where does the colour difference come from?
Addressing this question drove Shimomura's research during the 1960s and 70s to uncover the bioluminescent process of the jellyfish (Fig. 9).26 The process starts with the coelenterazine luciferin (2), a molecule from the imidazopyrazinone class (Fig. 3), reacting with oxygen to form a peroxide that, combined with the luciferase aequorin, forms a metastable adduct.2 This adduct is also known as a photoprotein, primarily serving as a reservoir of bioluminescence that is readily available to the jellyfish. Calcium ions trigger a conformational change in the aequorin, causing the opening of the imidazolone ring of the coelenterazine peroxy anion and the formation of a 1,2-dioetanone.2 The subsequent decomposition of this compound, releasing CO2, generates the excited state of oxyluciferin, also called coelenteramide, which emits light upon returning to its ground state. Therefore, unlike classical luciferin-luciferase reactions, jellyfish bioluminescence relies on the reaction of a luciferin and a protein conjugate with calcium ions, setting it apart mechanistically from other bioluminescent systems.2

Fig. 9. The mechanism of jellyfish bioluminescence. The precise protonation states are still uncertain2
Alongside the discovery of the photoprotein aequorin, Shimomura was able to isolate another protein in Aequorea victoria, GFP.1 This protein is what gives bioluminescent jellyfish their green, and not blue, glow.2 During a process called Forster resonance energy transfer (FRET), a chromophore enclosed within GFP absorbs the energy of the excited oxyluciferin. This elevates the chromophore to an excited state, from which it emits light.2 The energy released during GFP luminescence must be lower than that required for its excitation to comply with energy conservation laws. Since energy and wavelength are inversely proportionally related, a lower energy release leads to longer wavelength emission, hence the green light emitted by GFP.2
Currently, GFP is extensively used as a widely established and broadly applicable tool for fluorescent labelling in biological studies, such as tracking cancer tumours and investigating infectious diseases.9 In the artistic domain, several projects have emerged in the early 2000’s, such as the fluorescent genetically modified rabbit Alba2 by Eduardo Kac in collaboration with the geneticist Louis-Marie Houdebine.
Conclusions
Since the middle of the 19th century, much progress has been made towards understanding the chemistry behind bioluminescence in marine organisms, revealing fascinating and diverse processes. Modern analytical instruments have allowed researchers to uncover the structure of several luciferins and various chemical mechanisms are now well understood, opening the door to a wide range of applications. However, with the incredible diversity of life in the ocean, many bioluminescent species remain yet to be discovered and various processes still remain partially or completely unknown. These areas present researchers with exciting opportunities for future research.
References
- Haddock, S.H.D.; Moline, M.A.; Case, J.F., Annual Review of Marine Science 2010, 2, 443-493.
- Schramm, S.; Weiß, D., ChemBioChem 2024, 25, e202400106.
- Wilson, T.; Hastings, J.W., Annual Review of Cell and Developmental Biology 1998, 14, 197-230.
- Verdes, A.; Gruber, D.F., Integrative and Comparative Biology 2017, 57, 18-32.
- Widder, E.A., Science 2010, 328, 704-708.
- Markova, S.V.; Vysotski, E.S., Biochemistry (Moscow) 2015, 80, 714-732.
- Valiadi, M.; Iglesias-Rodriguez, D., Microorganisms 2013, 1, 3-25.
- World Hydrozoa Database, Aequorea victoria (Murbach & Shearer, 1902), World Register of Marine Species, https://www.marinespecies.org/aphia.php?p=taxdetails&id=283998 (accessed 02/06/2024).
- Kaskova, Z.M.; Tsarkova, A.S.; Yampolsky, I.V., Chemical Society Reviews 2016, 45, 6048-6077.
- Renwart, M.; Mallefet, J., Journal of Experimental Marine Biology and Ecology 2013, 448, 214-219.
- Nakamura, H.; Kishi, Y.; Shimomura, O.; Morse, D.; Hastings, J.W., Journal of the American Chemical Society 1989, 111, 7607-7611.
- Cormier, M.J.; Crane, J.M.; Nakano, Y., Biochemical and Biophysical Research Communications 1967, 29, 747-752.
- Claes, J.; Mallefet, J., Journal of Experimental Marine Biology and Ecology 2009, 51-65.
- Barnes, A.T.; Case, J.F.; Tsuji, F.I., Comparative Biochemistry and Physiology Part A: Physiology 1973, 46, 709-723.
- Frank, T.M.; Widder, E.A.; Latz, M.I.; Case, J.F., Journal of Experimental Biology 1984, 109, 385-389.
- Haddock, S.H.D.; Rivers, T.J.; Robison, B.H., Proceedings of the National Academy of Sciences of the United States of America 2001, 98, 11148-11151.
- AlgaeBase, Noctiluca scintillans (Macartney) Kofoid & Swezy, 1921, World Register of Marine Species, https://www.marinespecies.org/aphia.php?p=taxdetails&id=109921 (accessed 02/06/2024).
- Hanley, K.A.; Widder, E.A., Photochemistry and Photobiology 2017, 93, 519-530.
- Algaebase, Pyrocystis fusiformis C.W.Thomson, 1876, World Register of Marine Species, https://marinespecies.org/aphia.php?p=taxdetails&id=110328 (accessed 07/06/2024).
- World Ostracoda Database, Vargula hilgendorfii (Müller, 1890), World Register of Marine Species, https://www.marinespecies.org/aphia.php?p=taxdetails&id=450986 (accessed 02/06/2024).
- Shimomura, O.; Goto, T.; Hirata, Y., Bulletin of the Chemical Society of Japan 1957, 30, 929-933.
- Shimomura, O.; Johnson, F.H., Biochemical and Biophysical Research Communications 1971, 44, 340-346.
- Warner, J.A.; Case, J.F., The Biological Bulletin 1980, 159, 231-246.
- Oba, Y.; Kato, S.-i.; Ojika, M.; Inouye, S., Tetrahedron Letters 2002, 43, 2389-2392.
- Yuichi, O.; Shin-ichi, K.; Makoto, O.; Satoshi, I., Heterocycles 2007, 72, 673.
- Shimomura, O., The Biological Bulletin 1995, 189, 1-5.