Introduction
As the global economy attempts to reduce the effects of climate change by reducing its use of fossil fuels for energy production, it is turning to renewable forms of energy, generally produced as electricity. In turn, this increases the demand for minerals containing the metals needed for batteries in electric vehicles and to supply the relentlessly increasing number of information technology devices and their associated infrastructure.
“Rare earths are not that rare,” Marty Weems, the president for North America of American Rare Earths claimed in 2022, clarifying this by saying, “There are deposits of rare earths all over the world… The issue is finding them close enough to infrastructure, in places where a company can have all the permitting to operate, find the workforce to do the work, transport the material, and do all this in an economically viable way.”1
A promising process is using biosorption of rare earth elements (REE) on to E. coli,2 while an environmentally friendly and inexpensive adsorbent material using baker’s yeast and trimetaphosphate has demonstrated selective recovery of rare earth elements from hot springs and seawater.3
Although originally mined from deposits of Li- and REE-containing minerals,4 recent awareness of their high concentration in some geothermal waters has focused attention on the prospect of extraction of Li and REE from geothermal fluids and the siliceous sinters deposited from such fluids as they cool. Investigation of New Zealand’s geothermal fluids suggests that while the amounts of REE are variable between fields, there seem some prospects for REE recovery from them; this paper explores that possibility.
Cautionary tales
Lithium and the some of the so-called rare earth elements have recently risen to prominence in their technological applications. An example – now nearly ten years old – of REEs’ use was in taking advantage of the magnetic properties of two rare earths – neodymium (Nd) and dysprosium (Dy) – to enable the removal of gears from wind turbines.5 In 2013, the price of dysprosium oxide was US$ 1,508 per kg, and its current price is about US$ 370 per kg.6 As a metal, its variation in price from 2018 (US$ 238.14 per kg) to 2023 is shown in Fig. 1A;7 its current price is US$ 540.70 per kg. Neodymium shows a similar trend; the price of neodymium oxide in 2011 was US$ 250 per kg, and its current price is US$ 50 per kg.8 As a metal, its variation in price from 2018 (US$ 70.04 per kg) to 2023 is shown in Fig. 1B;9 its current price is US$ 128.40 per kg. From 2018-2023, Nd-prices are consistently about a quarter of Dy-prices. This would be expected, as neodymium is more abundant than dysprosium in the continental crust (the ratio of the concentration of the two elements in the earth’s crust is about 7 [Nd/Dy = 6.92]).10

The time trends for lithium prices provide a similar cautionary tale. Lithium is not rare, but was seemingly not important technologically until the development of Li-ion batteries, when it was found that using magnetite nanoparticles to capture Li showed promise."11 Following initial increases, the price of lithium has fallen dramatically recently (Fig. 2). On a more positive note, the technology used to recover lithium may be adapted to recover caesium.12

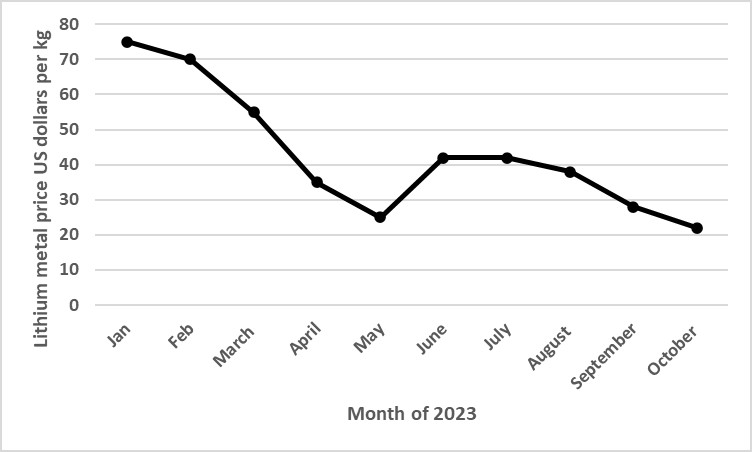
The ‘message’ from Figs 1 and 2 is that the markets for rare earth and other elements important for technology are highly volatile.
REE in New Zealand
Research into New Zealand deposits of minerals of potential economic value has included analytical data related to the rare earth elements. In a mid-1990s report, sources of REE minerals were identified in the South Island (and a map of their localities and some discussion of the minerals involved was included), but no specific connection with geothermal fluids was made.13 Some additional localities of REEs, including in Northland were added subsequently, but their origin was described in a Geological and Nuclear Sciences report as:
“Rare earths are known to occur in "carbonatite rocks" and "alkaline intrusions". In simple terms, they are magma chambers that rose towards the surface 40 million to 80 million years ago. They didn't quite reach the surface. Instead, numerous complex and rare processes occurred that concentrated the rare earth elements as the magma cooled and solidified underground.”14
This type of investigation led to a widespread – but erroneous – belief that REE were scarce, as recent commentary suggests:
“These metals are a critical component of magnets, fluorescent lights, video screens, catalysts, lasers, capacitors, optical glass and other high-tech applications. When they started running out, we were warned there would be critical shortages, a collapse of the technology industry, and perhaps war with China, the source of 95% of the world’s supply…. In reality, when China squeezed its exports in 2010 (not because of shortages but as a geopolitical and mercantile weapon) other countries started extracting rare earths from their own mines, recycling from industrial waste, and re-engineering products so they no longer needed them.”15
Potentially more relevant to New Zealand, REE are increasingly being found in geothermal areas around the world – in lakes associated with thermal springs or their sediments and in the geothermal fluids themselves.16 New Zealand is no exception. Indeed, as far back as 2006, a research report measuring the concentration of rare earth elements in a small number of New Zealand’s geothermal waters noted:
“Concentrations of rare earth elements (REE) in [a few] acidic thermal waters from the Taupo Volcanic Zone, New Zealand have been determined. Acidity in waters from Rotokawa results from oxidation of H2S upon encounter with oxygen-rich meteoric waters. The Ruapehu Crater lake is acidic due to input of magmatic volatiles such as HCl and SO2. REE concentrations in both types of water are high, ranging from 10− 3 to nearly 1 times chondrite.17 Chondrite-normalized patterns for heavy REE in waters from Rotokawa parallel those of their host rocks, suggesting little fractionation upon water–rock interaction. However, the light REE are depleted relative to their host rock, resulting in a ‘gull-wing’ pattern. The hyperacidic Ruapehu Crater lake water generally does not have a ‘gull-wing’ pattern….”18
At about the same time the abstract in a paper reporting the results of another study noted:
“…Using the rare earth element (REE) composition of the geothermal and surface waters, the competitive influences of mixing and water-rock interactions on fractionation were explored. Comparison of the REE composition of geothermal fluids, surface water, and sediments/ precipitates, indicate that temperature has a strong effect on REE fractionation in the sampled fluids. Eu- and Ce-anomalies (Eu/Eu*, Ce/Ce*) are visible in several samples and are related both to the inheritance of Eu and Ce abundances from geologic host materials and fractionation of these elements during water-rock interactions. The REE, trace element and major element data demonstrate that competitive processes, including water-rock interaction at depth with aquifer wall-rock, differential leaching of REE from mineral phases and precipitation of secondary minerals from geothermal fluids result in highly evolved fluids discharging into the surface waters of the TVZ [Taupo Volcanic Zone] ….”19
A decade later, in a US-based study, “no significant predictive relationship [of REE concentrations] to lithology, reservoir temperature, nor salinity was discovered.”20 Taken together with earlier data, the variability of these results suggest that concentrations for the assessment of REE ‘mining’ potential would be best determined from sampling deep geothermal fluids, as is done for recent data in Fig. 3.21

‘Mining’ of New Zealand’s geothermal fluids for REE
The concentrations of Li and the indicative REEs neodymium and dysprosium in the geothermal fluids, particularly when the ‘consented take’ (i.e., the amount of fluid allowed to be extracted annually) is taken onto account, from the geothermal fields currently being exploited in New Zealand are quite low (Table 1).22 Moreover, the currently low prices for Dy (Fig. 1), Nd (Fig. 1), and Li (Fig. 2) suggest that any economic benefit from ‘mining’ these metals alongside the current use of the fluid as the feedstock for electricity generation would be limited.

Economic and strategic considerations
If the price of the metals were to increase again to recent highs, i.e., a three-fold increase in the price of lithium (inferred from Fig. 2) , a two-fold increase in the price of neodymium, and a 1.5-fold increase in the price of dysprosium (the latter two inferred from Fig. 1), the ‘all-fields’ revenues calculated in Table 1 would increase to US$ 177,594,084 for lithium, to US$ 1,490,376 for neodymium and US$ 819,161 for dysprosium. Whether or not such yields are economic, the rare earth elements and lithium have the collective potential to be a strategic resource for New Zealand, either to bargain with countries, organisations or nations that already have refining facilities – or wish to have them – or perhaps to compete with friendly or even adversarial nations.
This would probably require collaboration between the landowners of the geothermal fields and the operators of the geothermal power stations in order to share facilities for recovery and possible refining of the extracted metals. This could be considered an ‘enlightenment’ phase of REE discovery, recovery and technological exploitation, akin to that which prevailed during investigations of the transport and deposition of precious and some base metals.23Legislatively, these developments might also require an appraisal of the current consenting process for ‘taking’ geothermal fluid. Both of these issues are discussed below.
Land ownership and operations
The historic connection of Māori with the geothermal resources has been included in many Waitangi Tribunal land claims, and for which there is also abundant evidence from many 19th century photographs that show them cooking (immersing kettles or flax kete in natural hot pools), as well as bathing in them, and guiding tourists around the naturally occurring thermal features (Fig. 4A-B). Māori appropriated guiding as a business, not only at thermal attractions owned by Māori, but also at those attractions leased to European operators (e.g. Orakeikorako); the business sometimes acquiring a cultural dimension by guides adopting ‘traditional dress’ or by their telling traditional Māori stories and legends (Fig. 4C-F).
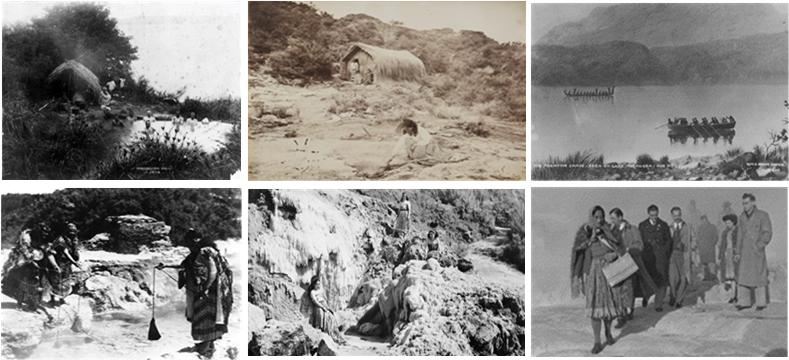
Examples of Māori tourism business: C, Upper right: The ‘phantom canoe’ claimed to be observed by Guide Sophia and allegedly seen by tourists just prior to the Tarawera eruption in 1886 (https://www.buriedvillage.co.nz/guide-sophia-hinerangi ). D, Lower left: Māori women, wearing semi-traditional clothing, cooking food in flax bags, using a thermal spring in Rotorua, probably in 1920s (Alexander Turnbull Library, PAColl-6585-43). E, Lower centre: The Golden Fleeces silica terraces, with Māori women alongside, in the1950s (Alexander Turnbull Library, 1/2-040319-F). F, Lower right: Unidentified members of a Bristol Freighter aircraft crew, with Guide Rangi, walking among hot thermal springs and bubbling mud pools, Rotorua, 1947 (Alexander Turnbull Library, WA-08419-f).
Until the 1990s, land ownership was not generally considered linked to the ownership of the geothermal resources beneath the surface, these resources being Crown-owned;24 negotiations in respect of the Mokai and Ohaaki-Broadlands geothermal fields started to change all that.25 Today most of the land above New Zealand’s geothermal resources is owned by Māori (Table 2), this change being succinctly expressed as:
“Under Te Tiriti o Waitangi, land and associated geothermal resources have been returned to iwi ownership and have been developed by land trusts and businesses for tourism, electricity generation and processing.”26
Under these new arrangements exploiters of the geothermal resource apply to the appropriate regional or district council for a resource consent to extract fluid (referred to here as ‘take’), a process broadly similar to the requirements for the extraction of water for farming, irrigation, etc.
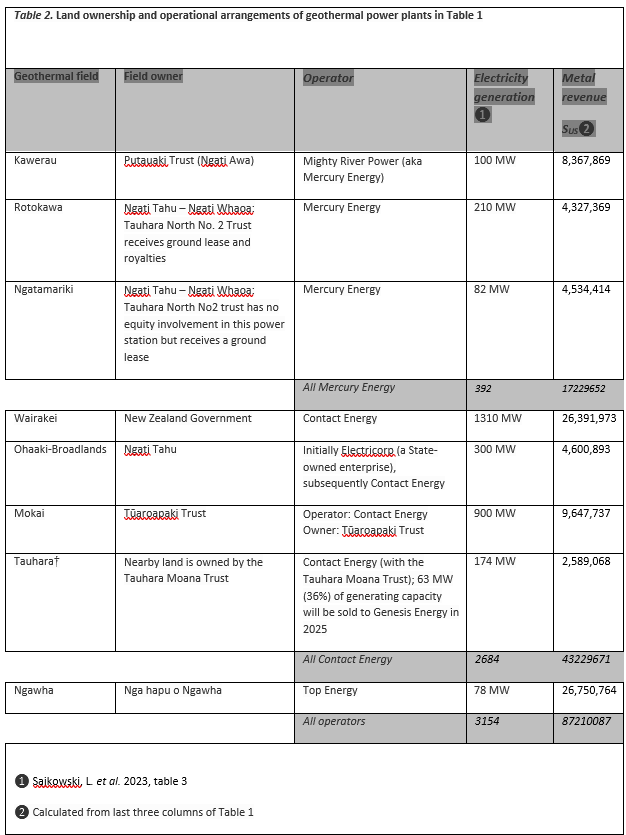
Wairakei Geothermal Field
Robert Graham purchased 4023 acres of the Wairakei Block in 1881, but not the adjacent Oruamuturangi Block. Both blocks were contentiously ‘purchased’ by the Government, leading to the comment: “Succeeding generations of Māori in the Taupo district have continued to question among themselves how Wairakei came to be ‘lost’.”27 A history of the development of the Wairakei geothermal field makes no reference to its contested ownership, simply observing that:
“The area at Wairakei was extensive with one of the largest estimated natural heat outflows in the thermal regions. The steam field and potential power station sites were on Crown land and the topography of the area, together with the availability of ample cooling water indicated a relatively straightforward power development. The proximity of the MOW [Ministry of Works] hydro project at Mangakino suggested a significant reduction should be possible in the time required to set up and begin work. Finally, the supply for the Wairakei Hotel was an encouraging sign regarding future drawoff from the field.”28
Kawerau Geothermal Field
“Putauaki Trust, a Māori Trust with over 500 shareholders owns and administers 1150 hectares of land in the Kawerau area with a significant portion of this land lying over the Kawerau Field geothermal resource.”29 Part of the land over the Kawerau Field was owned by the Crown which sold it to Mighty River Power in 2005, on the understanding that Mighty River Power would then on-sell the majority of these assets to Ngati Tuwharetoa Geothermal Assets Limited in a commercial deal. Ngati Tuwharetoa Geothermal Assets Limited, Norske Skog Tasman and Mighty River Power will also agree to a Steamfield Management Plan which will detail how the steam from the Kawerau field will be used.30 There are presently four developers on the Kawerau system, and this is permitted by the policies of the Waikato and Bay of Plenty Regional Councils, which is unusual on a world scale. While a multiplicity of developers can complicate and delay projects, it might foster the collaboration and flexibility suggested above.31 In 2025, in a similar way, 63MW of generating capacity of the Tauhara Geothermal field will be sold by Contact Energy to Genesis Energy in 2025.32
Ohaaki-Broadlands, Rotokawa, and Ngatamariki Geothermal Fields
Development of the Ohaaki-Broadlands, on Ngati Tahu -owned land and with private companies developing the field and operating the geothermal power station,33 was the precedent for similar arrangements for other geothermal fields in the Waikato – Bay of Plenty region. Initially Electricorp (a State-owned enterprise), operated the power station, but subsequently this became one of Contact Energy’s activities.34
Land owned by Ngati Tahu – Ngati Whaoa: Tauhara North No. 2 Trust overlies the Rotokawa and Ngatamariki geothermal fields, for which the owners have entered into a lease arrangement with Mercury Energy to develop the field and operate the power station. The Trust also receives royalties for the Rotokawa field.35
Mokai Geothermal Field
For this field, owned by the Tūaroapaki Trust, the development has proceeded rather differently: “The trust was formed by 297 families amalgamating their land in the 1950s, initially for beef and sheep farming and later for geothermal power. The Tūaropaki Trust owns the power station and contracted its operations initially to Mighty River Power” – now Mercury Energy.36
Tauhara Geothermal Field
In a similar approach to that of the development at Ohaaki-Broadlands “… the Tauhara Moana Trust who own land adjacent to where the power station is being built… have a commercial agreement in place to allow for the utilisation of the geothermal resource beneath the Trust’s land and for the Trust to farm all of Contact’s land on the Tauhara field. If geothermal energy is found under the Trust’s land it will be used in the power station and a portion of the profits will go to the Trust. Contact [Energy] continues to look to foster long-lasting relationships with landowners to develop further renewable generation projects.”37 As mentioned in the section on the Kawerau Geothermal Field, ownership of part of the generating capacity of the Tauhara field will be transferred from Contact Energy to Genesis Energy in 2025.
Ngawha Geothermal Field
This field, centred on the Northland township of Ngawha, and the site of an historic bathing facility, is owned by Nga hapu o Ngawha.38
Future prospects
Unless there are significant changes in demand for REE that result in higher prices, only the mining of New Zealand’s geothermal resources for lithium seems economically feasible, with the ‘major players’ in such an activity being Contact Energy (at Wairakei, Ohaaki-Broadlands, Mokai, and Tauhara) and Top Energy (at Ngawha). By combining the metal operations across the geothermal fields on which Mercury Energy operates (Kawerau, Rotokawa, and Ngatamariki) it could be considered a ‘minor player’ in geothermal mining.
If the annual ‘take’ of geothermal fluid were to be increased, the amount of lithium and REE potentially recoverable would obviously increase. However, increasing the ‘take’ simply to foster metal recovery seems unlikely. More probable is the recognition that electricity generated from solar and wind is unlikely to be sufficient to meet the normal household and business needs of New Zealand’s growing population as well as meeting the nation’s international obligations to reduce greenhouse gas emissions by converting the vehicle fleet from using fossil-fuels to using ‘green’ electricity. Moreover, if there was an excess of electricity generated it could be used to electrolyse water, the hydrogen being sold domestically to replace natural gas. Increasing the ‘take’ of geothermal fluid from existing fields – or new ones – would serve these political and environmental ends, and might also enable a subsidiary metal extraction industry.
Finally, there are always compromises. Firstly, geothermal power stations do generate greenhouse gases, but no more than would have been emitted naturally,39 and, furthermore, those gas emissions are about a quarter of that released from the burning of fossil fuels.40 Secondly, even with reinjection41 and other technologies designed to extend the life of geothermal fields during high-volume fluid extraction, the lifetime of those fields will inevitably be reduced. The Wairakei field provides a useful example: the chloride concentration in wells drilled in the late 1950s was about 2000 ppm; over the following 16 years the chloride concentration reduced to 876 ppm.42 Extrapolation of the plot of chloride versus time for the 70 years since withdrawal started (Fig. 5) tentatively predicts that the chloride concentration would now be about 8% of the original concentration. However, the actual concentration is much higher (1991 ppm43) than that because other wells have been drilled nearby – notably in the Te Mihi field – to augment the declining supply of geothermal fluid from the original wells.44 With or without the mining of metals from the geothermal fluid, this augmentation scenario seems likely to be replicated in other high-producing geothermal fields in the future.

log (C/Co)= -0.0161*t + 0.0406
For t = 70 years, this gives a value of C/Co of ~0.08
Notes and references
- Dettoni, J. Rare earths are not that rare. FDI Intelligence, 13October, 2018. https://www.fdiintelligence.com/content/interview/rare-earths-are-not-that-rare-81560 (accessed 20/10/2023).
- Brewer, A.;Chang, E.; Park, D.M. et al. Recovery of rare earth elements from geothermal fluids through bacterial cell surface adsorption’ Environmental Science and Technology, 2019, 53(13),7714-7723, https://doi.org/10.1021/acs.est.9b0030
- Kihara, M.; Ojima, Y.; Azuma,M. Adsorption of rare earth ions from synthetic seawater and hot spring water using phosphorylated yeast. Environmental Technology and Innovation 2023, 30(6), http://dx.doi.org/10.1016/j.eti.2023.103093
- The Chinese mining of rare earths has been described as “a very dirty business. Rare earths are often found with radioactive elements like thorium and uranium, and separating them out requires a lot of toxic chemicals’, referring to Jack Lifton, founder of Technology Metals Research and an expert on rare earths, description of how, “in China, the process of extraction involves leaching out the elements. They flood the high ground with chemicals, he says, and then precipitate out the metals, leaving behind a lake of carcinogenic waste fluids.” From: Rowlatt, J. (2014, March 23). Rare earths: Neither rare, nor earths. 23 March 2014. https://www.bbc.com/news/magazine-26687605 (accessed 20/10/2023).
- Rowlatt, 2014, op. cit.
- Dysprosium oxide price worldwide from 2009 to 2020 with a forecast for 2021 to 2030. https://www.statista.com/statistics/450164/global-reo-dysprosium-oxide-price-forecast/ (accessed 17/10/2023).
- Dysprosium Historical Price Movement. https://strategicmetalsinvest.com/dysprosium-prices/#:~:text=The%20current%20price%20of%20Dysprosium%20is%20%24540.70%20per%20kg (accessed 17/10/2023).
- Neodymium oxide price worldwide from 2009 to 2020 with a forecast for 2021 to 2030. https://www.statista.com/statistics/450152/global-reo-neodymium-oxide-price-forecast/ (accessed 17/10/2023).
- Neodymium Historical Price Movement. https://strategicmetalsinvest.com/neodymium-prices/ (accessed 17/10/2023).
- Abundance of Nd in upper continental crust is 27 ppm; Dy 3.9 ppm cited In Handbook on the Physics and Chemistry of Rare Earths, 2016, Section 2.2.1 ‘Bulk silicic Earth’.
- How PNNL Extracts Rare Earth Elements from Geothermal Brine, Water Network Research. 2021. https://thewaternetwork.com/article-FfV/how-pnnl-extracts-rare-earth-elements-from-geothermal-brine-VSi63Uw-1pc_wy89G7tUiQ (accessed 17/10/2023).
- “Geo40 has developed innovative world-leading technology for the recovery of strategic minerals from geothermal brines. A sustainable future relies upon sustainable sources of future minerals; recovering silica from geothermal fluid opens up new opportunities in the geothermal ecosystem. We have built a pioneering team to tackle the climate-critical challenges that we face in the road ahead. Our world-first, commercial Northern Plant processes 6,700 tonnes of geothermal fluid per day and will recover up to 5,000 tonnes per annum of colloidal silica from New Zealand geothermal brine. colloidal silicas, we are particularly motivated by markets where our silica can displace environmentally unsustainable alternatives, such as polymers, which invariably end up in waterways…” https://geo40.com/ (accessed 17/10/2023).
- Christie, T.; Brathwaite, B.; Tulloch, A. Mineral Commodity Report 17 - Rare Earths and Related Elements. New Zealand Petroleum and Minerals, 2000. https://www.nzpam.govt.nz/assets/Uploads/doing-business/mineral-potential/rare-earths.pdf (accessed 17/10/2023).
- Harvie, W. Rare earth could be common in NZ. 4 November 2018. https://www.stuff.co.nz/science/108283898/rare-earth-could-be-common-in-nz (accessed 17/10/2023)\.
- Pinker, S. Enlightenment Now. Penguin, United Kingdom, 2018, pp. 126-127. As an aside, Pinker notes that such a response to an alleged shortage of resources is not unique: the so-called ‘peak oil’ crisis is another example: the use of natural gas as an alternative to petroleum and the development of electricity generation from the sun, wind and tides can be seen as ways by which this apparent crisis has been averted.
- Smith, Y.R.; Kumar, P.; McLennan, J.D. On the extraction of rare earth elements from geothermal brines. Resources 2017, 6 (3), 39. https://doi.org/10.3390/resources6030039
- These values are higher than those in Wood, S.A.; Shannon, W.M. Rare earth elements in geothermal waters from Oregon, Nevada and California. Journal of Solid State Chemistry 2003, 171 (1-2), 246-253. “Concentrations of REE range from <10-6 to approximately 10-3 times chondrite... In general, filtered aliquots of the fluids contain substantially less REE (sometimes by an order of magnitude or more) than corresponding unfiltered aliquots, suggesting a considerable particulate contribution to the total REE.”
- Wood, S.A. Rare earth element systematics of acidic geothermal waters from the Taupo Volcanic Zone, New Zealand. Journal of Geochemical Exploration 2006, 89 (1), 424-427.
- Hannigan, R.E. Rare earth, major, and trace element geochemistry of surface and geothermal waters from the Taupo Volcanic Zone, North Island New Zealand. In Rare Earth Elements in Groundwater Flow Systems, edited by Johannesson, K.H., Water Science and Technology Library, vol 51. Springer, Dordrecht, 2005. https://doi.org/10.1007/1-4020-3234-X_3 (accessed 17/10/2023).
- Hannigan’s conclusion contrasts with: Quillinan S.; Nye. C.; Engle, M. et al. Assessing rare earth element concentrations in geothermal and oil and gas produced waters: A potential domestic source of strategic mineral commodities (Final Report),University of Wyoming, Laramie, 2018, for which the abstract notes: “The project team collected and analyzed 224 water samples and 101 matching rock samples… [Its] improved method of measuring aqueous REEs allows study of samples previously thought too volume-limited to measure. The study found that aqueous REEs occur at trace levels in all analyzed samples, and sometimes exceed ocean REE concentrations by a factor of 1,000. No significant predictive relationship to lithology, reservoir temperature, nor salinity was discovered, but aqueous REE concentration appears spatially controlled.”
- Sajkowski, L.; Turnbull, R.; Rogers, K. A review of critical element concentrations in high enthalpy geothermal fluids in New Zealand. Resources 2023, 12 (6), 68. https://doi.org/10.3390/resources12060068. Crustal composition data from: Kumari, A.; Panda, R.; Jha, M.K.; Kumar, J. Process development to recover rare earth metals from monazite mineral: A review. Minerals Engineering 2015, 79, http://dx.doi.org/10.1016/j.mineng.2015.05.003
- Sajkowski, L. et al. 2023, op. cit. table 3.
- Hodder, P. Salt and gold: mining the geothermal resource in New Zealand. Chemistry in New Zealand 2016 80 (1), 27-32.
- Bennion, T. New Zealand Law and the Geothermal Resource Waitangi Tribunal WA1200, A018.
- White, B.; Morris, G.; Lumb, J.T. New Zealand Geothermal Resource Ownership – Cultural and Historical Perspective. International Geothermal Association, mid-1990s [inferred], pp. 575-580 https://www.geothermal-energy.org/pdf/IGAstandard/WGC/1995/1-white.pdf (accessed 24/10/2023).
- New Zealand’s history of geothermal energy. GNS Science. https://www.gns.cri.nz/our-science/energy-futures/geothermal-energy/new-zealands-history-of-geothermal-energy/ (accessed 24/10/2023).
- Stokes, E. Wairakei Geothermal Area: Some Historical Perspectives. University of Waikato, Hamilton, 1991, pp. 21-78.
- Bolton, R.S. Notes on the early history of Wairakei. Proceedings 20th New Zealand Geothermal Workshop, 1998.
- Putauaki Trust. https://www.putauakitrust.com/geothermal/ (accessed 17/10/2023).
- Crown agrees to Kawerau geothermal assets deal. https://www.beehive.govt.nz/node/23671 (accessed 17/10/2023).
- Lawless, J.; van Campen, B.; Randle, J. Future Geothermal Generation Stack. A report for the Ministry of Business, Innovation and Employment, 2020, p. 12. This report also commented, “The over-production and consequential severe decline of the Geysers geothermal resource in the USA was a consequence of multiple tapper access, which was only resolved when one single operator effectively remained in operation and took steps to recover the reservoir pressure through supplementary injection.”
- Long-term PPA signed for Tauhara geothermal project, NZ. https://www.thinkgeoenergy.com/long-term-ppa-signed-for-tauhara-geothermal-project-nz/ (accessed 17/10/2023).
- Stokes, E. A power station on Maori land. Te Matahauariki Institute, University of Waikato, Hamilton, 2003.
- Later operational information from: Ohaaki Geothermal System. New Zealand Geothermal Association. https://www.nzgeothermal.org.nz/geothermal-in-nz/nz-geothermal-fields/ohaaki/ (accessed 24/10/2023).
- Tauhara North No. 2 Trust. Geothermal ngawha. https://tauharano2.co.nz/commercial/geothermal/ (accessed 17/10/2023).
- Legmann, H.; Citrin, D. First twelve months of operation of the 60 MW Mokai Geothermal Project: A high pressure, sustainable and environmentally benign power plant. Proceedings of the 5th INAGA Annual Scientific Conference and Exhibitions, 2001. See also: https://tuaropaki.com/business/geothermal-power-generation/ (accessed 17/10/2023).
- Tauhara – building a better future. https://contact.co.nz/aboutus/our-story/our-projects/tauhara (accessed 17/10/2023).
- The Ngawha Geothermal Resource Report, Waikato Tribunal Report WAI 304. Legislation Direct, Wellington, 1993.
- Cariaga, C. Geothermal power plants have zero impact on GHG emissions, affirms study. Think Geoenergy, 2021. https://www.thinkgeoenergy.com/geothermal-power-plants-have-zero-impact-on-ghg-emissions-affirms-study/ (accessed 17/10/2023).
- Energy Efficiency and Conservation Authority (EECA) asserts: “While electricity generated from geothermal energy produces some greenhouse gas emissions, the average emissions intensity of geothermal electricity is about one-quarter of that of the cleanest natural gas-fuelled power station.” https://www.eeca.govt.nz/insights/energys-role-in-climate-change/renewable- energy/geothermal/#:~:text=Low%20emissions%20%E2%80%93%20While%20electricity%20generated,natural%20gas%2Dfuelled%20power%20station (accessed 17/10/2023).
- Choudhary, A.; Burnell, J., Rayudu, R.; Hinkley, J. Greenhouse gas emissions from geothermal powerplants in New Zealand: Is reinjection of gases a solution? IEEE Region 10 Annual International Conference Proceedings/TENCON TENCON 2021 - 2021 IEEE Region 10 Conference (TENCON), 2021, pp. 811-815. https://doi.org/10.1109/TENCON54134.2021.9707393 (accessed 17/10/2023).
- Glover, R.B. Chemical and physical changes at Geyser Valley, Wairakei, and their relationship to changes in borefield performance. In Geochemistry 1977, compiled by A.J. Ellis, edited by A.P.W. Hodder, DSIR Bulletin 1977, 218, 19-30.
- Sajkowski et al. 2023, op.cit., Table 2.
- Thain, I.A.; Carey, B. Fifty years of geothermal power generation at Wairakei. Geothermics 2009, 38 (1), 48-63.