Abstract
Terry Seward (1940 – 2022) was a prominent scientist in the field of hydrothermal fluid geochemistry. This paper analyses the variation with time of the citations of Terry Seward’s publications, the references he used, and the language he used in his abstracts. Citation analysis reveals that his papers have a longer influence on his area of research than is typical, but, more significantly, demonstrates the adoption of Terry Seward’s chemistry findings (many of which were published in Geochimica et Cosmochimic Acta) by other scientists, and published subsequently in the geological literature. This, and his record of co-authorship, provides clear evidence of Terry Seward’s influence within and beyond his particular field of research. The dominant positivity of language Terry Seward generally used in his abstracts may have assisted this process. An interesting feature of his research is that a significant proportion of his later publications of mechanisms for the transport and deposition of metals are vapour-based (reminiscent of the idea proposed by the medieval scientist René Descartes) rather than ‘hydrothermal’ or hot-water/solution-based (reminiscent of the idea proposed by the medieval metallurgist Georgius Agricola). This is not to imply a change in Terry Seward’s preferring one mechanism over another, but, rather, a broadening of his perspective on a controversy having its origins in the Middle Ages, which probably facilitated his final engagement with a new research initiative – the ‘Geothermal: The Next Generation’ project – focused on much deeper and hotter geothermal resources than had been explored and exploited to date.
Introduction
When precious metals and base-metal sulfides were recovered from the ‘scale’ of geothermal wells,1 the relative concentrations of precious and base metals in the scale was higher than would have been expected on the basis of the sulfides’ low solubility in water and this prompted interest in the mechanism by which the metals could be transported and deposited. Initial suggestions about the mechanism by which the metals could be transported and deposited favoured the precipitation of gold, silver and base metal sulfides from aqueous metal complexes, typically involving sulfur species or chloride,2 and many laboratory investigations have been undertaken that demonstrate this suggestion.3 As one example, the solubility of gold in the system H2O-H2-HCl-NaCl-NaOH at temperatures of 300-600oC and pressures of 500-1800 bar was “accurately described” by Eq. 1:4
Au(0)(s) + 2 Cl- (aq) + H+ (aq) = Au(I)Cl2- (aq)+ 0.5 H2 (gas) (Eq. 1)
The ability of gold to dissolve under these conditions enables it to be carried as the complex ion (Au(I)Cl2-) in solution, only to be re-deposited elsewhere when the temperature, pressure, and other conditions are suitable. Fig. 1A and Fig. 1B show the results of some examples of experimental work on gold complexes,5,6 while Fig. 1C shows the variation of the stability of zinc complex ions with pH.7

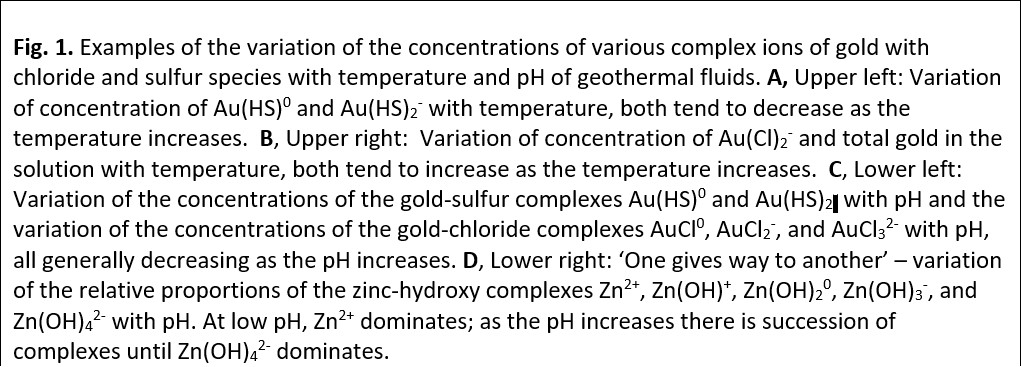
Experiments on one metal have enabled inferences to be drawn about another. An example is an investigation of the solubility of nickel in solutions of increasing temperature and/or chloride concentration which both identified and determined the formation constants of NiCl+, NiCl2(aq) and NiCl3- at temperatures up to 700oC and pressures up to 2000 bar,8 the report further noting a comparison with the behaviour of cobalt: “The solubility of millerite (NiS) and pentlandite (Ni4.5Fe4.5S8) calculated using these constants shows that nickel dissolves in significantly higher concentrations in hydrothermal solutions than previously estimated. However, the solubility is considerably lower than for corresponding cobalt sulfide minerals. This may explain why hydrothermal nickel deposits are encountered so much less frequently than hydrothermal deposits of cobalt.”
Comparisons between experimental data for metals and their natural occurrence have also been undertaken for gold and silver.9 This study suggested that gold chloride species were more abundant than bisulfide species in fluids responsible for auriferous deposits, but bisulfide species were more abundant than chloride species in fluids responsible for Au-Ag deposition. This latter conclusion was subsequently supported by Liu et al (2013);10 a similar conclusion having been earlier reached for lead and zinc.11 Laboratory experiments are but proxies for reality: a paper by Bodner (2004), quaintly entitled “Heavy metals or punk rocks?”, observing in its abstract: “Most ore geologists now agree that fluids with metal contents sufficient to produce economic mineralisation are relatively common and that it is the lack of a suitable depositional mechanism that often limits ore formation. Temperature decrease alone cannot be the dominant mechanism, because the solubility of most metals in most hydrothermal fluids decreases by only a small amount over the temperature range determined for most deposits. Thus, other processes – such as boiling or immiscibility, fluid mixing, or fluid-rock interactions – must operate to promote the precipitation of all (or most) of the dissolved metals transported by hydrothermal fluids.”12
The involvement of a high-temperature vapour phase as part of the mechanism for metal transport and deposition in the hydrothermal environment had been suggested a few years earlier by Hedenquist and Lowenstern (1994), who considered that: “Magmatic fluids, both vapour and hypersaline fluid are a primary source of many components in hydrothermal ore deposits formed in volcanic arcs. These components, including metals and their ligands, become more concentrated in magma in various ways and from various sources, including subducted oceanic crust. Leaching of rocks also contributes to the hydrothermal fluid – a process enhanced where acid magmatic vapours are absorbed by deeply circulating meteoric waters.”13
Experiments to test this idea’s prospects have been undertaken.14 One specific example investigated the solubility of copper chloride in liquid under-saturated HCl-bearing water vapour at temperatures of 280-320oC and pressures up to 103 bar, for which a hydrated copper-chloride gas species was formed (Eq. 2):15
3 Cu(I)Cl(s) + n H2O(gas) = Cu(I)3Cl3(H2O)n (gas) (Eq. 2)
However, the earlier described notion of transport in solution still has strong support, Williams-Jones et al (2005) noting, “Despite increasing evidence to the contrary, most economic geologists still assume that hydrothermal ore deposits form exclusively from aqueous liquid.”16
In fact, there is nothing new in this debate between the mechanisms of metal transport involving liquid and gaseous phases; it recalls the difference between Agricola’s contention in the mid-1500s that ores are derived from percolating groundwater, and Descartes’ view formulated a century later that ores are derived from magma. Georgius Agricola’s (1494 - 1555) 1556 book, entitled De Re Metallica, included a classification of ores based on genesis, which was a strong foundation for later theories on ore deposits. Centuries later, Ball (2015) observed of Agricola’s ideas: “Agricola supposed that mineral veins are formed when groundwater percolating through the rocks, carrying dissolved salts is ‘baked by subterranean heat to certain denseness’ and so forms metal ore deposits. ...recouched in modern terms, his idea becomes a demonstrable truth: many ores are formed when groundwater dissolves minerals in the soil and rock and then precipitates them elsewhere in new combinations of elements.”17 René Descartes (1596-1650), in his 1644 book (initially written in Latin and re-published in French in 1647) entitled Principia Philosophiae, believed that ore minerals were driven upward from a deep metalliferous shell by interior heat in the form of exhalations and rising surface waters to be deposited as lodes in the fissures of the Earth’s outer stony crust. The Italian scientist Nicolaus Steno (1638 -1686) developed this idea further and in 1669 proposed that ores are a product of condensation from vapours ascending through open fissures. These two contrasting ideas are discussed succinctly in Skinner (1973).18
As is often the case, it seems that it’s not a question of ‘either – or’ but of ‘both’. Thus, it is likely that for precious and base metals that either or both of solution transport (i.e., the Agricolan preference) and vapour transport (i.e., the Descartesan preference) can operate, albeit under different conditions. Those conditions include whether the reactions are occurring in an aqueous liquid or a gas medium, or something in between, i.e., a fluid (fluids have properties intermediate between a liquid and a gas in a similar way that a glass has properties intermediate between a solid and a liquid). This will influence whether a chemical species exists as a simple charged ion, a complex ion (e.g., Au(I)Cl2- in Eq. 1), or an uncharged molecule (e.g., Cu(I)3Cl3(H2O)n(gas) in Eq. 2).
Geological inferences from the hydrothermal model
A leading scientist in unravelling the transport of metals in fluids was Professor Terry Seward, a fragment of whose extensive research is referred to above. Transport of metals as complexes and deposition from these complexes as pressures and temperatures decrease has been the dominant mechanism used to show how “active geothermal systems such as Ohaaki Broadlands and Rotokawa ... can transport and deposit significant quantities of gold... At Rotokawa, sulfide-rich surface muds contain up to 50 ppm gold and are still being deposited”.19 Towards the end of the twentieth century New Zealand scientists recognised the chemical and geological similarity between active geothermal systems, e.g., Wairakei and Broadlands, and the ‘old’ gold deposits of the Coromandel Peninsula, comparing them, as in Clark and Williams-Jones 1990, or by illustrating them side by side as in Moore and Ritchie (1996),20 or asserting: “High-temperature geothermal systems are the modern analogue of epithermal precious metal ore deposits. In these systems, gold and silver are transported primarily as bisulfide complexes, with the precious metals being deposited in response to boiling and mixing of the deep geothermal fluid.”21
Figs. 2 and 3 show the distribution of elements and their ions studied by Seward and his associated researchers, for which Railsback’s (2018) form of the chemists’ periodic table has been used – one that emphasises the distribution of similar ions rather than the distribution of the ‘neutral’ chemical elements.22 This seems appropriate as the complex ions in hydrothermal solutions are dependent on the charge (z) and the radius (r) the metal ions involved, from which the ‘ionic potential’ (the ratio z/r) can be calculated.23 From both Fig. 2 and Fig. 3 it is clear that the so-called ‘intermediate cations’ (those which have “at least a few outer-shell electrons, as in Fe2+ ”)22 have dominated Seward’s research on metal complexes in geothermal systems.

Fig. 2. Ions in complexes referred to in the titles and/or abstracts of Terry Seward’s publications, shown on the skeletal framework of the ‘cation’ side of the ‘Earth scientist’s periodic table of the elements and their ions’ (Railsback 2018, p. 209, Fig. 11.1).The number in parentheses beneath the symbol of the element is its ‘ionic potential’, the ratio of the charge on the ion (z) to its radius (r), i.e.,(z/r). The colours used on the diagram define ranges of ionic potential shown below:

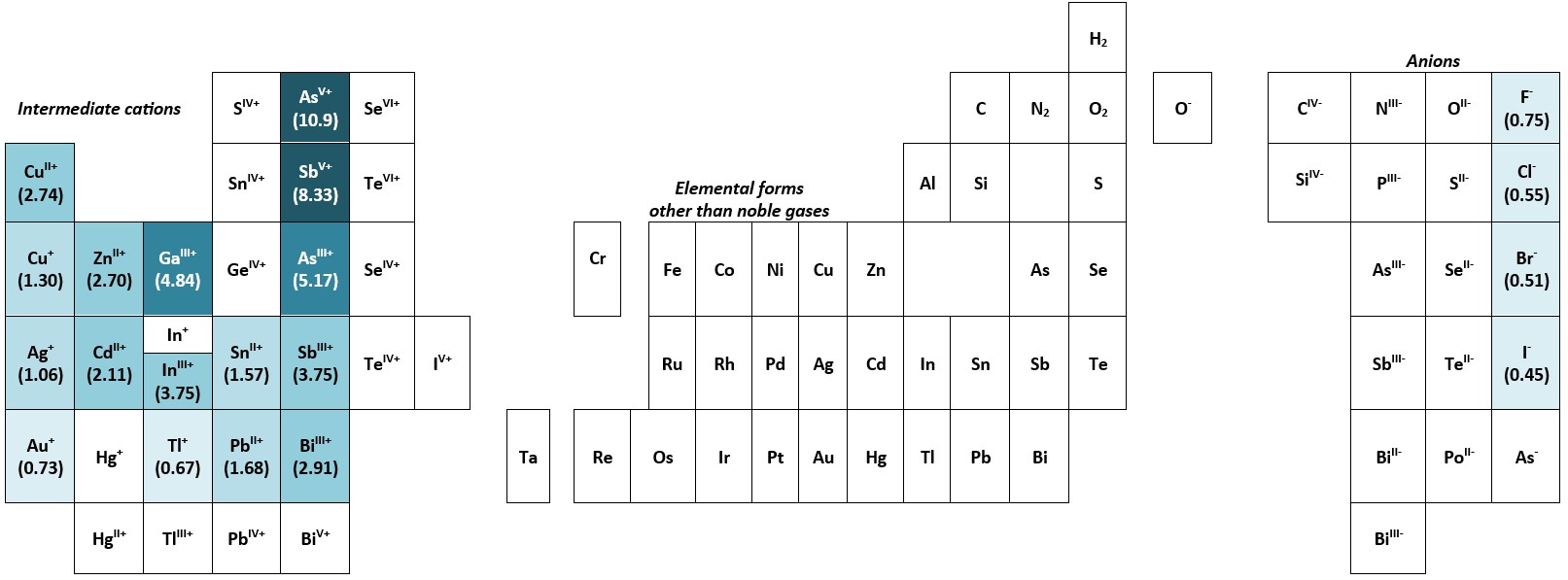
Fig. 3. Ions in complexes referred to in the titles and/or abstracts of Terry Seward’s publications, shown on the skeletal framework of the ‘anion’ side (excluding the noble gases helium, He; neon, Ne; argon, Ar; krypton, Kr; xenon, Xe; and radon, Rn) of the ‘Earth scientist’s periodic table of the elements and their ions’ (Railsback 2018, p. 210, Fig. 11.2). The number in parentheses beneath the symbol of the element is its ‘ionic potential’, the ratio of the charge on the ion (z) to its radius (r), i.e., (z/r). With the exception of SiIV-, the colours used on the diagram define ranges of ionic potential shown below:

Citation analysis
Scientific papers invariably include a list of references to previous research which the author has used to confirm or assist in the development of the research that the paper describes. Some of the readers of a paper will mention the author’s work (i.e., they ‘cite it’) in their own papers and will include it – together with other research – in the list of references within their papers. Usually the number of citations of a paper reaches a maximum a couple of years after publication, the citations generally being made by scientists in a similar line of research.24 However, sometimes scientists in quite a different field of research notice the paper, read it, and cite it in their own work. Several of Terry Seward’s papers which were not extensively cited25 show the classic maximum number of citations after just a few years (Fig. 4 A-D),26 but some of his papers continue to be cited – and even show a resurgence – a decade after their publication (e.g., Fig. 4E-F).27 This ‘double maximum’ suggests that some of his papers have found a new audience. How this could have come about is explained below.

All of Seward’s cited papers included in Fig. 4 are chemically oriented, those in the left-hand diagram of Fig. 4 have their maximum number of citations about five years after publication (especially curves B and C). On the right-hand diagram of Fig. 4, curve D reaches its maximum within a year of publication and declines thereafter. Perhaps because of the paper’s title – which includes the mineral name, sphalerite (zinc sulfide), Fig. 4F appears to have the potential to reach a geological audience: its citation curve takes three years to achieve its first maximum, thereafter maintaining a variable but high number of citations. Fig. 5 shows that the articles citing the paper shown for curve F of Fig. 4 were predominantly published in chemistry journals initially, but later citations are mainly in geology journals. In a similar way, curve E in Fig. 4 may appear initially to be complicated, but the variation of the number of citations with time could be inferred to be a combination of an initial citation curve generated from the interest shown by chemists followed – or overlapped – by a citation curve generated from the interest shown by geologists.
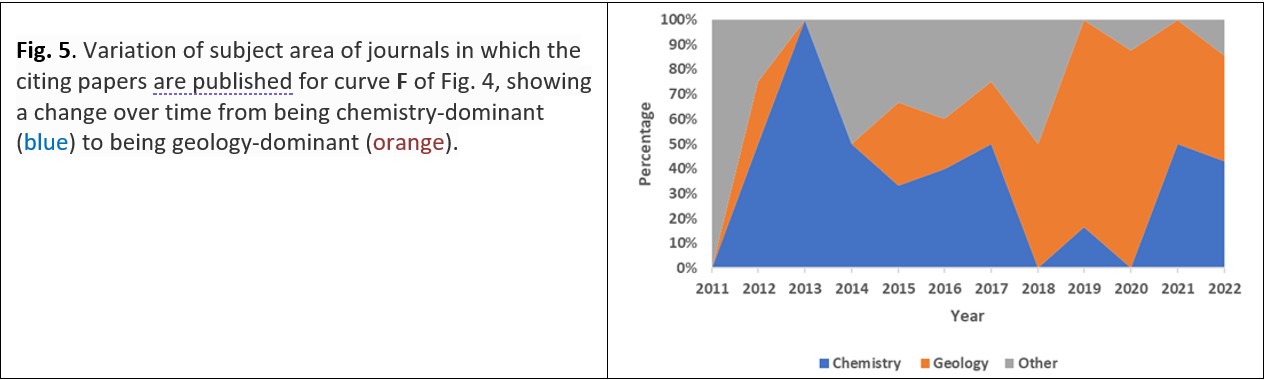
This finding of a new audience is particularly apparent in the citation curves for two of Seward’s more highly cited papers (Fig. 6).28 In these instances the papers have been found of interest to geologists as well as chemists, and geological journals feature strongly in the citations.

Curve A of Fig. 6 shows a maximum of annual citations five years after publication which is largely attributed to chemists citing the research by Seward and his colleagues; the subsequent two maxima of annual citations in 2019 and 2022 – about a decade later – are dominated by geologists’ citations (in 2019, only 4% of the citing papers were published in chemistry journals while in 2022, a fifth (20%) of the citing papers were published in chemistry journals). Curve B of Fig. 6 – for a publication published in a geologically-themed journal – shows the second maximum in 2020 even more clearly: only 6% of the citing journals are chemistry-themed. Across all of Terry Seward’s publications this secondary peak a few years after the initial peak is just apparent above the ‘noise’ (Fig. 7).29

By this process ideas are transferred from one scientific discipline to another and interdisciplinary research thrives. Seward himself may have helped to foster these trends because some of his journal articles – particularly later in his career – were actually published in geology-themed journals, and so would have been seen by geologists rather than chemists (Fig. 8).29

Citations of a paper can be supportive of the idea proposed in it (i.e., positive), opposed to the idea (i.e., negative), or simply observing its existence, but not specifically supporting or opposing it; with Cohn and Farrington (1994) noting that most citations are generally positive or neutral.30 A sample of the citations of Seward’s most cited paper (Seward 1973, with over 800 citations)31 accords with this finding, with 66% being positive, 26% being neutral, and 9% being negative. There is also a variation with time, as shown on Fig. 9.
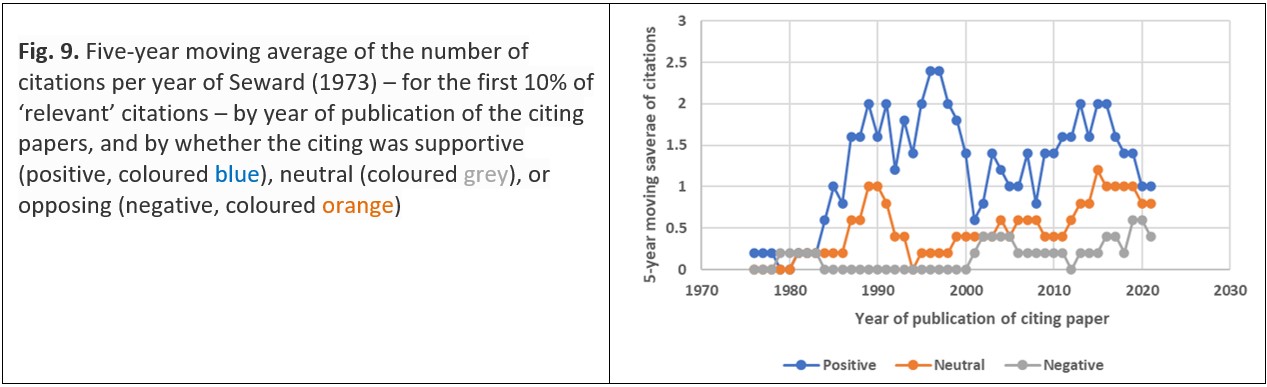
The number of citations supporting the idea overall show a decrease with time since the cited paper was published; while the number of citations opposing or simply observing the idea’s existence increase with time since the cited paper was published. However, the most noticeable feature of Fig. 9 is the decrease in supporting citations from 2000 to 2010. This is most probably caused by an increased publication of papers seeking explanations for ore deposition in geological settings other than those associated with geothermal systems and is consistent with the increase in citations of Seward’s geochemistry in geologically themed journals referred to earlier in this paper. In that context an increase in the number of citations might be seen as a measure of ‘impact’, at least within an academic or professional community. Another explanation for at least some of the variation seen in Fig. 9 is Aksnes’ (2005) identification of ‘citing as a social process’ – “to recognise one’s friends and network acquaintances”.32 As Warrall and Cohn (2023) point out, the use of such measures in defining research quality is problematic,33 and, accordingly, no measure of Terry Seward’s research quality is inferred from citation analysis in this paper.
Sentiment analysis and measures of collaboration
Sharing ideas has long been an important characteristic of scientific research, and citation is an important component of that sharing, especially when the researcher who is doing the citing works in a different organisation (or even a different country) from the researcher who wrote the paper that is being cited. Sharing ideas can also be fostered by the author who uses language in the paper that resonates with the reader: sentiment analysis of the text is one technique by which this resonance could be measured. Sentiment analysis was developed to track the emotion in fictional stories, especially in novels,34 but is now frequently used in marketing. In Table 1 and Fig. 10, a readily available free software package was used to determine the sentiment of the abstracts of Seward’s scientific publications;35 the applicability of which to ‘fact-based’ text has been demonstrated by Byrne et al. (2021).36 The ‘sentiment’ or ‘emotion’ of a piece of text can be positive, negative or neutral as determined by an integrated or holistic analysis of words and their context in a piece of text. Table 1 shows three examples from Terry Seward’s papers: an abstract of positive sentiment, an abstract of neutral sentiment, and an abstract of negative sentiment,37 with words and phrases highlighted that contribute to – but do not themselves define – the sentiment polarity determined by the software.
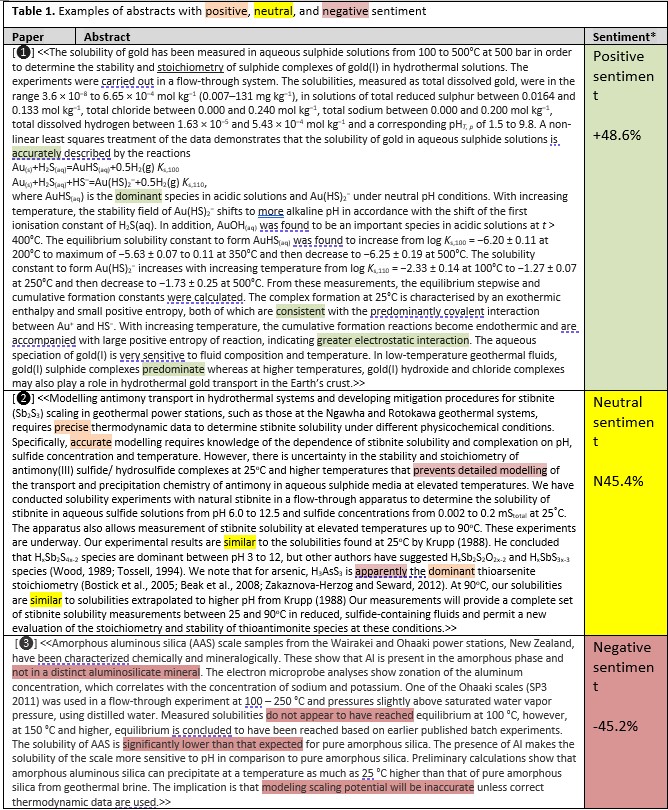

For Terry Seward’s papers, there is no significant trend for abstracts of his publications that have positive sentiment to increase as the number of citations increases, but there is a rather more pronounced trend for abstracts with negative sentiment to become less negative as the number of citations increases (Fig. 10A). There is also an overall trend of the sentiment of Terry Seward’s abstracts becoming more positive with time (Fig. 10B), although it is unclear whether this can be attributed to a deliberate effort by him or a change in specification of abstracts by the journal in which he published (most frequently Geochimica et Cosmochimica Acta).

With the growth of online publication of research and the widespread availability of online searching of research, concern has been expressed that innovation in science might be impaired: “… as more journals come online, the article references tend to be more recent, fewer journals and articles are cited and most of those citations were to fewer journals and articles. The forced browsing of print archives may have stretched scientists and scholars to anchor findings deeply into past and present scholarship. Searching online is more efficient and following hyperlinks quickly puts researchers in touch with prevailing opinions, but this may accelerate consensus and narrow the range of findings and ideas.”38 In the specific example of Terry Seward’s publications, although the number of references becomes more variable with time and the number increases towards the present (Fig. 11), this trend does not support the suggestion made earlier in this paragraph.
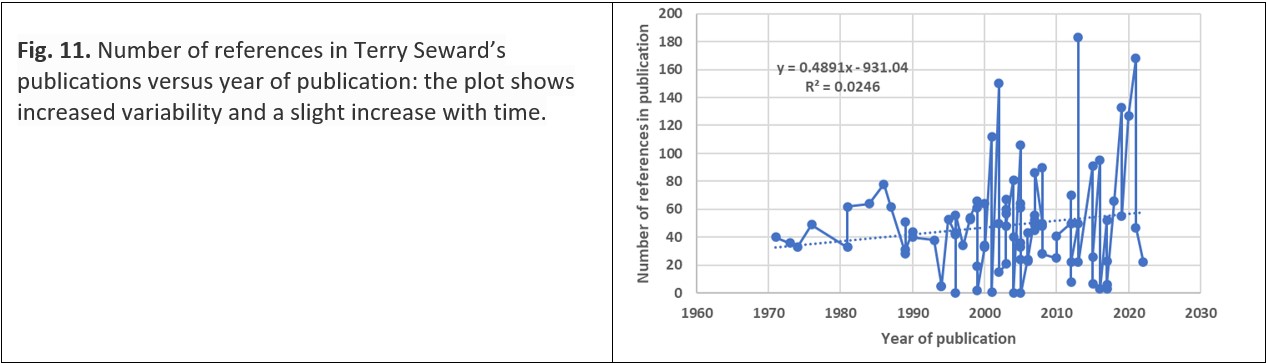
Another way of sharing ideas is through co-authorship. Researchers on the staff of universities will often prepare a paper based – either wholly or partly – on the research undertaken by their master’s or doctoral student, with the student typically involved in drafting the paper. Students who move on to other institutions to undertake postdoctoral study or employment may continue to collaborate – in both research and writing – with their former supervisors. In addition, researchers based in universities and other research-based organisations (e.g., the Crown Research Institutes in New Zealand) may choose to collaborate with researchers who are either within or outside their own institution and who have similar research interests; these collaborators may, in turn, collaborate with others and so the network of researchers in a particular area expands. Thus, a given researcher may have a network of collaborative relationships with many – perhaps dozens or even hundreds – of other researchers. In common with many researchers, as Terry Seward’s career progressed there were more collaborative publications and fewer publications of which the researcher is the sole author (Fig. 12).
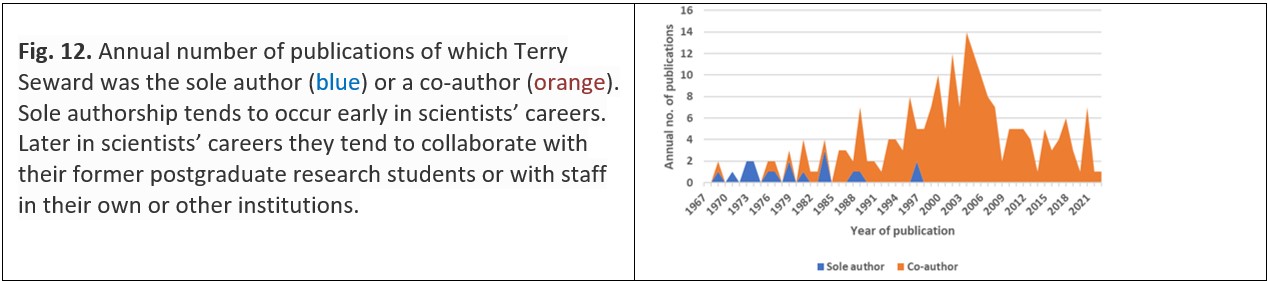
Co-authorship, in which several scientists and/or their students participate, can result in large groups of people involved in the preparation of a single paper, particularly where the research is itself a group project – usually caused either by the amount of work envisaged by the project leaders or by the cost of specialist equipment or facilities. Such projects are often the successful outcome in a competitive application for large research grants, generally managed by large quasi-government agencies. By comparison, the size of Seward’s groups were modest (Fig. 13). For a variety of reasons (including personal preference or perceived accrual of professional advantage) some researchers are more collaborative than others. Although one of Seward’s co-authors collaborated with him on 16 publications, typically co-authorship involves fewer publications than this, as shown in Table 2. Dissemination of research results through publishing either by co-authorship (as described above) or citation (as described earlier) is complemented by researchers attending specialist and general meetings and conferences,39 and – more recently – participating in webinars and other similar activities.

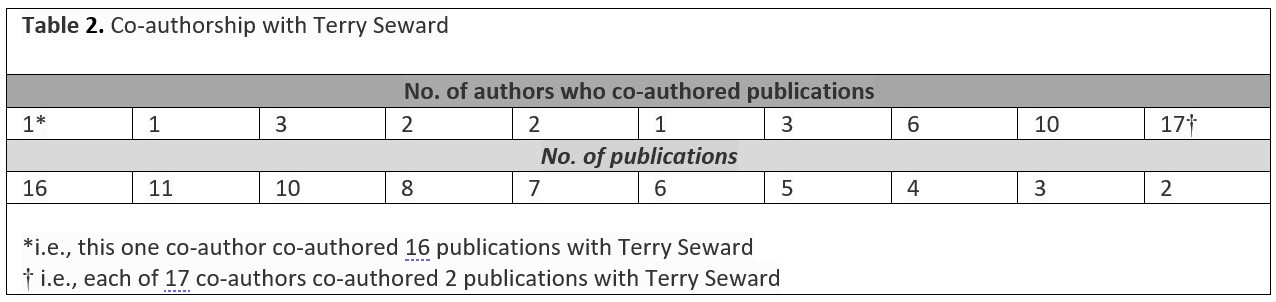
A ‘turn’ towards vapour transport
More recently Terry Seward turned his thoughts to the importance of vapours and gases in the “complexing” and transport of metals in steam and low density supercritical water.40 His response to a question about its significance was that this ‘turn’ in his research had resulted in a paper which: “… presents new and hitherto unavailable data on the molecular structure of steam and low density supercritical water and solutions. Of particular interest are our data on the pH of steam and low density supercritical water, which is the subject of only a handful of papers in the world of chemistry/physics literature. Low density supercritical water is composed of nanodroplets or water clusters, the size of which is defined by the temperature and pressure (or density). We demonstrated that the solvated proton (or hydronium ion [H3O+] if you prefer) sits on the surface of these water clusters, thus emphasising the reactivity of low density supercritical water/solutions. This is quite a different scenario compared to liquid water, in which ionic species (e.g., the proton) are “internally” solvated in the hydrogen bonded dielectric continuum that is water liquid.”41
Consistent with this ‘turn’ (which by no means implies that solution transport no longer has relevance),42 simulations of reactions at supercritical conditions have been undertaken (e.g., Passarella et al., 2017)43 and their wider geological implications explored, as by Henley and Seward (2018).44 An interesting feature of the distribution over time of Terry Seward’s publications is the change in the relative number of publications about metal transport under hydrothermal conditions, where the solution temperature is typically lower than 350⁰C and the pressure less than that corresponding to a depth of 3.5km (i.e., an ‘Agricolan’ mechanism) to the prospect of metal transport in the vapour phase (i.e., a ‘Descartesan’ mechanism) as might occur in volcanic gases and perhaps in a ‘critical fluid’ (in the case of water of temperature higher than 400⁰C and a pressure corresponding to a depth greater than 5km). Fig. 14 shows that although hydrothermal studies continue to dominate Seward’s research, his more recent research has started to broaden: it includes papers with a theme of the transport of metals via vapour transport in volcanic gases and/or involving a critical fluid, generally associated with magma (i.e., molten rock). This plot provides an example how a researcher’s focus or ideas can change with time. Such a change may be influenced by the collaborative processes described above, or it may be because new opportunities for research arise. For Terry Seward, the change may be partly associated with his late-career involvement with the ‘Geothermal: the Next Generation’ project, with its focus on chemical and physical processes occurring at greater depths – and, thereby, higher temperatures and pressures – than those to which much of his research had earlier been directed,45 but it also could imply a continuation of the quest for an enhanced understanding of ore-forming processes that has been a puzzle since medieval times.

Conclusion: Terry Seward as a geochemical ‘hero’
The journey of a hero has been recently recast in terms of seven ‘elements’ (Fig. 15), and is said by its proponents to offer as a “powerful way to reframe experiences”.46 This approach to describing Terry Seward’s academic and professional life is shown in Table 3.


Fig. 15. The many steps of a “hero’s journey”, described by mythologist Joseph Campbell,47 have been recently abridged to just seven key ‘elements’, viz.,1, protagonist; 2, shift; 3, quest; 4, allies; 5, challenge; 6, transformation; 7, legacy.46 The purple images on this diagram (modified from the original circular graphic) are examples of the translation of classic notions of these ‘elements’ (yellow images) into modern-day equivalents.

Notes and references
- Brown, K. 1986. Economic Geology 1986, 81, 979-983.
- Barnes, H.L., Rose, A.W. Science. 1998, 279 (5359), 2064-2065.
- Stefánsson, A.; Seward, T.M. Geochimica et Cosmochimica Acta 2004, 68 (20), 4121-4143; Tagirov, B.R.; Suleimenov, O.M.; Seward, T.M. Geochimica et Cosmochimica Acta 2007, 71 (20), 2436-2447; Tagirov, B.R; Seward, T.M. Chemical Geology 2010, 269 (3-4), 304-311.
- Stefánsson, A.; Seward, T.M., Geochimica et Cosmochimica Acta 2003, 67 (23), 4559-4576.
- Williams-Jones, A.E.; Bowell, R.; Migdisov, A. Elements 2009, 5 (5), 281-287.
- Wang, S-X.; Williams-Jones, A.E.; Bi, X-W.; Jiang, Z-Q.; Shang, L-B. 2022. Ore Geology Reviews 2022, 149, article no. 105091.
- Likholyl, A.; Hovey, J.K.; Seward, T.M. Geochimica et Cosmochimica Acta 2005, 69 (12), 2949-2958.
- Liu, W., Migdisov, A.; Williams-Jones, A. Geochimica et Cosmochimica Acta 2012, 94, 276-290.
- Shikazono, N.; Shimizu, M. Mineralium Deposita 1987, 22, 309-314.
- Liu, W.; Etschmann, B.; Testemale, D.; Mei, Y.; Hazeman, J-L.; Rempel, K.; Müller, H., Brugger, J. Mineralogical Magazine 2013, 77 (5, Goldschmidt Conference Abstracts), 1627.
- Stoffell, B.; Williamson, J.; Jeffries, T. American Journal of Science 2004, 304 (6), 533-557
- Bodner, R.J. Science 2004, 323 (5915), 724-725.
- Hedenquist, J.W.; Lowenstern, J.B. Nature 1994, 370 (6490), 519-527.
- Examples include: Heinrich, C.A.; Driesner, T.; Stefánsson, A.; Seward, T.M. Geology, 2004, 32 (9), 761-764; Zhang, S.; Xiong, X.; Seward, T.M. Earth Science Frontiers 2009, 16 (1), 68-75; Migdisov, A.A.; Williams-Jones, A.E. Geochimica et Cosmochimica Acta 2013, 104, 123-135.
- Archibald, S.M.; Migdisov, A.A.; Willliams-Jones, A.E.. Geochimica et Cosmochimica Acta 2002, 66 (9), 1611-1619.
- Williams-Jones, A.E.; Heinrich, C.A.; Migdisov, A.A. Goldschmidt Conference Abstracts 2005. Vapor and the Transport of Metals, 2005, 733.
- Ball, P. H2O: A Biography of Water, Weidenfeld and Nicholson, London, 2015, 43.
- Skinner, B.J.; Barton, P.B. Annual Review of Earth and Planetary Sciences 1973, 1, 813-211.
- Stefánsson, A.; Seward, T.M. Geochimica et Cosmochimica Acta 2004, 68 (20), 4121-4143.
- Clark, J.R.; Williams-Jones, A.E. Nature 1990, 346, 644-645; Moore, P.; Ritchie, N. Coromandel Gold: A guide to the Historic Goldfields of Coromandel Peninsula, Dunmore, Palmerston North, 1996, 21-27, fig. 2.1.
- Brown K.L.; Simmons, S.F. Geothermics 2003, 32 (4), 619-625.
- Railsback, L.B. The earth scientist’s periodic table of the elements and their ions. In Mendeleev to Oganesson: A Multi-disciplinary Perspective on the Periodic Table (Eds.: Scerri, E.; Restrepo, G.), Oxford University Press, New York, 2018, 206-218. See also: https://railsback.org/PT.htm
- Ionic radii in Fig. 2 and Fig. 3 are mostly from Krauskopf, K.B.; Bird, D.K. Introduction to Geochemistry. 2nd edition. McGraw-Hill, New York, Appendix VI, 1995, 595-597.
- Hodder, A.P.W.; Balog, C. Journal of Information Science 1984, 9, 221-226; Parolo, P.D.P.; Pan, R.K.; Ghosh, R.; Huberman, B.A. et al. Journal of Informetrics 2015, 9, 734-745.
- Compiled from: Publications – Terry Seward. https://www.wgtn.ac.nz/sgees/about/staff/staff-publications/publications-terry-seward (accessed October-November 2023).
- Likholyl, A. et al. Geochimica et Cosmochimica Acta 2005, 69 (12), 2949-2958; Stefánsson, A., et al. Proceedings of ICPWS XV (15th International Conference on the Properties of Water and Steam), Berlin, 2008; Zakaznova-Herzog, V.P., Seward, T.M. Geochimica et Cosmochimca Acta 2006, 70 (9), 2298-2310; Zakaznova-Herzog V.P., et al., Geochimca et Cosmochimca Acta 2006, 70 (9), 1928-1938.
- Tagirov, B.R. et al. Geochimica et Cosmochimica Acta 2007, 71 (20), 4942-4953; Tagirov, B.R, Seward, T.M. Chemical Geology 2010, 269 (3-4), 304-311.
- Stefánsson, A., Seward, T.M. Geochimica et Cosmochimica Acta 2004, 68 (20), 4121-4143; Heinrich, C.A. et al. Geology 2004, 32 (9), 761-764.
- Compiled from: Terry Seward – Google Scholar, https://scholar.google.com/citations?user=A6zwzl4AAAAJ&hl=en (accessed 10-14 Dec 2023).
- Cohn, E.G., Farrington, D.P. British Journal of Criminology 1994, 34 (2), 204-225.
- Seward, T.M. Geochimica et Cosmochimica Acta 1973, 37 (3), 379-399. The sample used is the first ten pages of the online compilation, sorted by ‘relevance’.
- Aksnes, D.W. Citations and Their Use as Indicators of Science Policy. Studies of Validity and Applicability Issues with a Particular Focus on Highly Cited Papers. Doctoral Thesis, University of Twente, 2005.
- Worrall, J.L.; Cohn, E.G. Journal of Contemporary Criminal Justice 2023, 39 (3), 327-340.
- Jockers, M. 2014. A novel method for detecting plot. https://www.matthewjockers.net/2014/06/05/a-novel-method-for-detecting-plot/ (accessed 04/03/2024).
- https://monkeylearn.com/sentiment-analysis-online/ (accessed 06/02/2024).
- Byrne, M. et al. PLOS One 2021, 16 (12), e0259797.
- Mountain, B.J. et al. NACE Corrosion Conference, 2013, NACE-2013-2246; Olsen, N.J. et al., New Zealand Geothermal Workshop Proceedings, 2012, 19-21 November 2012, Auckland; Stefánsson, A., Seward, T.M. Geochimica et Cosmochimica Acta 2004, 68 (20), 4121-4143.
- Evans, J.A. Science 2008, 321 (5887), 395-399.
- A historic example of this is detailed in: Uglow, J. The Lunar Men: The Friends Who Made the Future 1730 – 1810. Faber and Faber, London, 2002.
- Lemke, K.H.; Seward, T.M. Reviews in Mineralogy and Geochemistry 2018, 84, 57-83.
- Team profile: Terry Seward. In: Geothermal: The Next Generation. https://www.geothermalnextgeneration.com/updates/team-profile-terry-seward (accessed 08/02/2024).
- Seward, T.M.; Williams-Jones, A.E.; Migdisov, A. In Treatise on Geochemistry, Volume 13 Geochemistry of Mineral Deposits (Eds: Holland, H.; Turekian, K.) Elsevier, 2013, 29-57; Simmons, S.F.; Brown, K.L.; Tutolo, B.M. Economic Geology 2016, 111 (3), 589-618.
- Passarella, M.; Mountain, B.; Seward, T.M. Procedia Earth and Planetary Science 2017, 17, 770-773.
- Henley, R.; Seward, T.M. Reviews in Mineralogy and Geochemistry 2018, 84 (1), 309-349.
- Geothermal: the Next Generation. https://www.gns.cri.nz/research-projects/geothermal-the-next-generation/ (accessed 08/02/2024).
- Rogers, B.; Gray, K.; Christian, M. Scientific American 2023, 330 (1), 82-83; Rogers, B.; Chicas, H.; Kelly, J.M. Journal of Personality and Social Psychology 2023, 125 (4, October), 725-778.
- Joseph Campbell (1904-1987) was an American author and editor who examined the functions of myth in various human cultures. His best known work is: Campbell, J. The Hero with a Thousand Faces. Pantheon Books, New York, 1949. For a critique of Campbell’s approach to scholarship see: Lefkowitz, A. The American Scholar 1990, 59 (3), 429-434.
- Roberts, A.C.; Hawthorne, F.C. The Canadian Mineralogist 2002, 40 (4), 1191-1198.
- The 2005 Robert Wilhelm Bunsen Medal was awarded to Terry Seward for his pioneering contributions in hydrothermal geochemistry, particularly the development and application of in situ techniques to determine compositions and structures of hydrothermal solutions. https://www.egu.eu/awards-medals/robert-wilhelm-bunsen/2005/terry-m-seward (accessed 08/03/2024).